Chapter 8: Elimination Reactions
8.1 E2 Reactions
8.1.1 E2 Mechanism
The E2 mechanism is the bimolecular elimination mechanism, and the reaction rate depends on the concentration of both the substrate and base. We will take the elimination reaction of 2-bromo-2-methylpropane as an example for discussion.
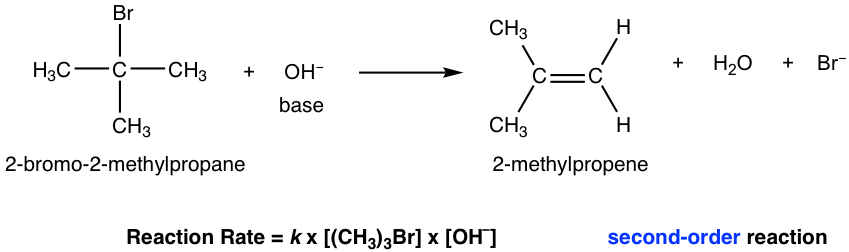
The E2 mechanism is also a single-step concerted reaction, similar to SN2, with multiple electron pair transfers that happen at the same time.
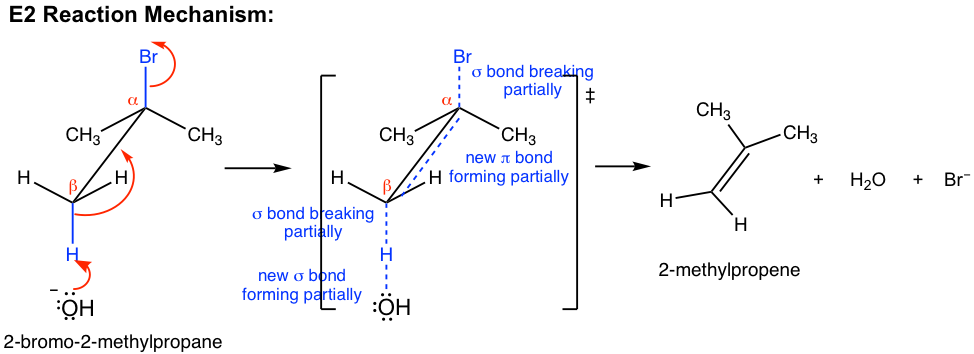
The base, OH–, uses its electron pair to attack a β-hydrogen on β-carbon and starts to form a bond; at the same time, the β C-H σ bond begins to move in to become the π bond of a double bond, and meanwhile, Br begins to depart by taking the bonding electrons with it. A transition state is formed in the reaction process with partially breaking and partially forming bonds. At the completion of the reaction, the C=C double bond and H2O molecule are fully formed, with Br– leaving completely.
Since both the substrate (halide) and the base are involved in the single-step mechanism, E2 is the second-order reaction.
8.1.2 Regioselectivity of E2 reaction: Zaitsev’s Rule vs Hofmann’s Rule
For the reaction we talked about in the above section, there are three β-carbons in the substrate 2-bromo-2-methylpropane; however, they are all identical so the reaction gives only one single elimination product: 2-methylpropene.
For other alkyl halides, if there are different β-carbons in the substrate, then the elimination reaction may yield more than one product. For example, the dehydrohalogenation of 2-bromo-2-methylbutane can produce two products: 2-methyl-2-butene and 2-methyl-1-butene by following two different pathways.
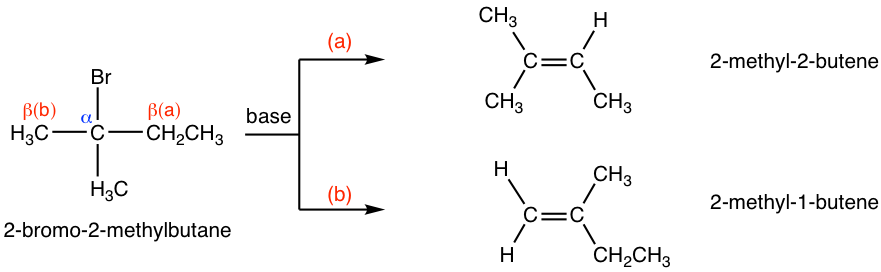
Between the two possible products, 2-methyl-2-butene is a trisubstituted alkene, whereas 2-methyl-1-butene is monosubstituted. For alkenes, the more alkyl groups bonded on the double bond carbons, the more stable the alkene is. Generally, the relative stability of alkenes with a different number of substituents is:
tetrasubstituted > trisubstituted > disubstituted > monosubstituted > ethene
Therefore, 2-methyl-2-butene is more stable than 2-methyl-1-butene. When a small-sized base is used for the elimination reaction, such as OH–, CH3O–, or EtO–, the relative stability of the product is the key factor in determining the major product. As a result, 2-methyl-2-butene is the major product of the above reaction.
As a general trend, when a small base is applied, the elimination products can be predicted by Zaitsev’s rule, that said the more substituted alkene is obtained preferably. So, Zaitsev’s rule essentially can be explained by the higher stability of the more substituted alkenes.
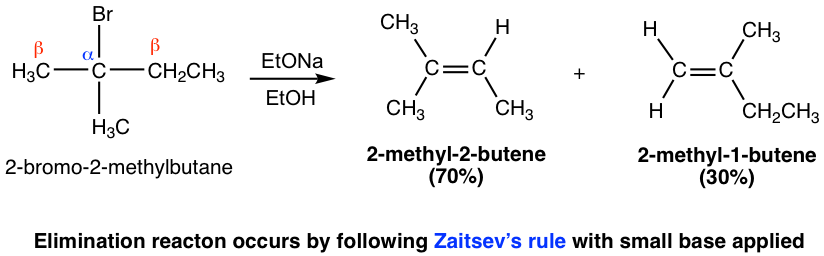
However, if a bulky base is applied in the elimination, such as t-BuOK, the reaction favors the formation of less substituted alkenes.
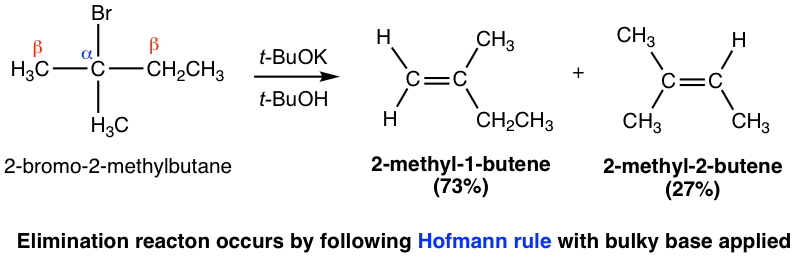
This is mainly because of steric hindrance. With t-BuO– attacking the β-hydrogen, it is difficult for this big, bulky base to approach the hydrogens from the β-carbon that is bonded with more substituents (as shown in the pathway (a) below), while the hydrogen of the methyl group is much easier to access (in the pathway (b) instead). When the elimination yields the less substituted alkene, it is said that it follows Hofmann’s rule.
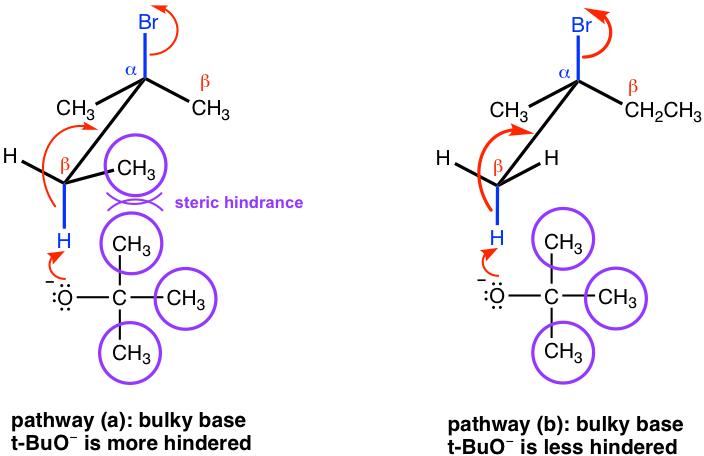
8.1.3 Stereochemistry of the E2 Reaction
The E2 mechanism has special stereochemistry requirements to ensure it proceeds. First, the bond connected with the leaving group and the bond connected with the H must be in the same plane to allow the proper orbital overlapping of the two carbons in the formation of the π bond of the alkene product. Second, the leaving group and H must be in anti-position to each other. This is because the anti-position allows the transition state of the reaction to be in the more stable staggered conformation, which helps to lower the energy level of the transition state and speed up the reaction. Overall, the E2 reaction proceeds with the leaving group, and H is in an anti-coplanar conformation.
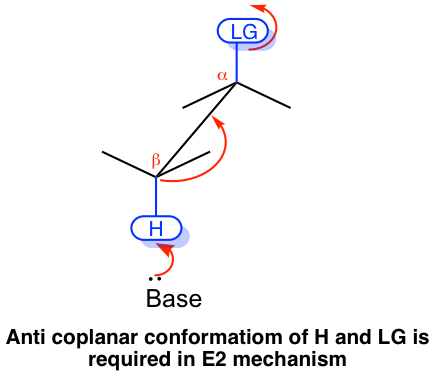
Because of the anti-coplanar conformation requirement for the E2 reaction, one stereoisomer will be produced preferably over the other, and this is called stereoselectivity. For the following example, the elimination of (2S,3S)-2-bromo-3-phenylbutane produces the E isomer specifically, not the Z isomer at all. This is because when H is in an anti-position to the leaving group Br, the whole compound is in a staggered conformation, and the other groups retain their relative position in elimination which leads to the E isomer.
Exercises 8.1
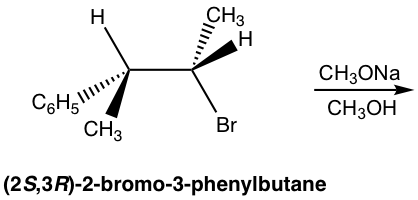
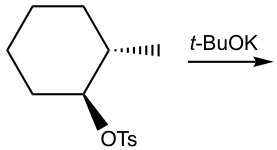
Answers to Chapter 8 Practice Questions
8.1.4 Bases in E2 Reactions (Brief Summary)
The most commonly applied bases in an E2 reaction are hydroxide OH– and alkoxide RO–. Specifically, the combination of a base with the corresponding alcohol is used broadly, such as CH3ONa/CH3OH or C2H5ONa/C2H5OH.
Examples of small bases: OH–, CH3O–, C2H5O–, and NH2–
Examples of big bulky bases: t-BuO– and LDA (lithium diisopropylamide) with the structures shown below.