Chapter 10: Alkenes and Alkynes
10.8 Alkynes
Alkyne is a hydrocarbon that contains a C≡C triple bond. In this section, we will explore methods for the synthesis of alkyne and the chemical reactions of alkynes.
10.8.1 Acidity of Terminal Alkynes and Related Reactions
In the discussions of acids and bases (Chapter 3), we have learned that the hydrogen atom bonded to the terminal alkyne carbon shows higher acidity than the hydrogen atoms bonded to the carbons of an alkene or alkane, and the pKa value of the terminal alkyne hydrogen is about 25.
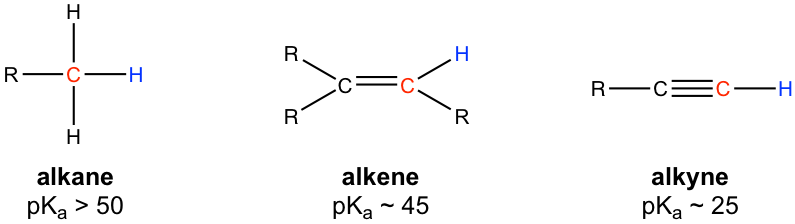
Because of the relatively high acidity, the terminal alkynes can be deprotonated by appropriate strong bases, such as NaH, and NaNH2.

The product of the above deprotonation, alkynide anion, is a good nucleophile that can be used in an SN2 reaction with primary substrates (since primary substrates work best for such SN2 reactions as we have learned):

A new carbon portion is introduced in the product with a new carbon-carbon bond formed in the SN2 reaction, and this is a common method to synthesize internal alkynes with longer carbon chains. A specific example of the synthesis of 2-methyl-3-hexyne from 3-methyl-1-butyne is given here:
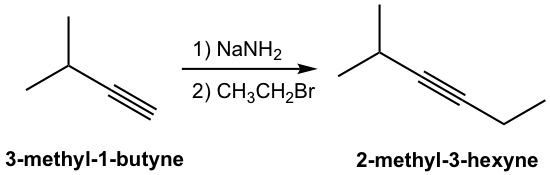
The method in 10.4.1 applies to the synthesis of alkyne with a certain structure. The more general way to synthesize alkyne is via the elimination reaction of vicinal dihalides. Recall that vicinal dihalides are the halogenation products of alkenes (section 10.4). The vicinal dihalide can then be subjected to a double dehydrohalogenation reaction with a strong base to produce an alkyne.
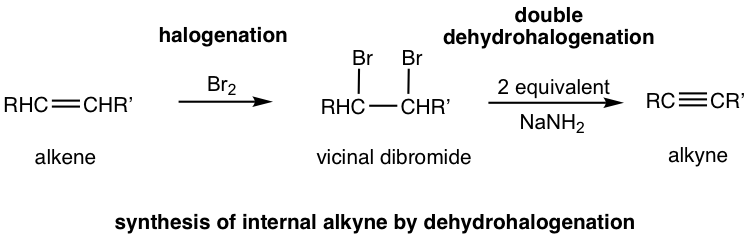
The dehydrohalogenation occurs twice, in two steps. The first product is a haloalkene, and the second product is the alkyne. Amide, usually NaNH2, is a base that is strong enough to cause both reactions carried out consecutively in the same mixture. Two molar equivalents of sodium amide per mole of the dihalide are required to ensure that the elimination occurs two times.
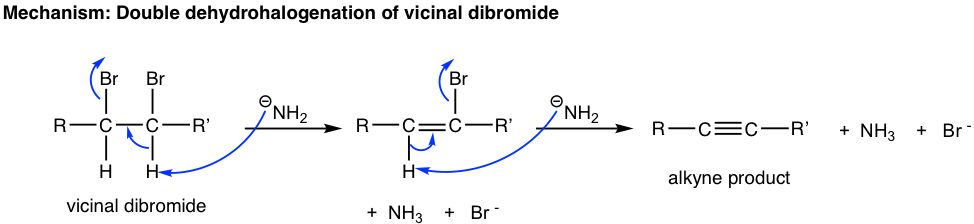
If a terminal alkyne is the desired product, then three molar equivalents of a base are required. The terminal alkyne produced after double dehydrohalogenation is deprotonated by sodium amide, and the third mole of the base is to ensure that the deprotonation occurs completely and all the terminal alkynes convert to the salt format. The salt of alkynide was then treated with ammonium chloride (or water, as a source of protons) to produce terminal alkyne as the final desired product.
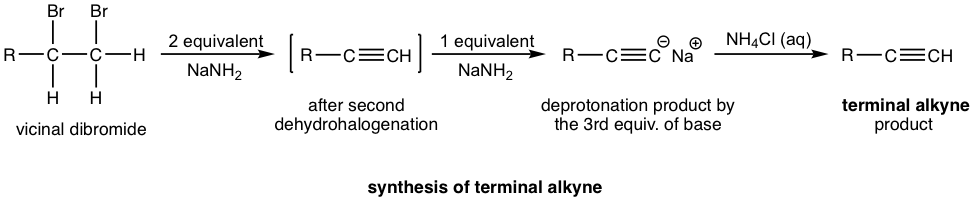
Examples
Design the synthesis route of 1-butyne from 1-butene.
Approach:
Use retro-synthetic analysis:
That analysis can be translated to the step-by-step synthesis as:
Solution: (show the steps together in the proper order, without showing intermediates for each step)
10.8.3 Reactions of Alkynes
Hydrogenation of Alkynes
Catalytic hydrogenation applies to the π bonds of C≡C triple bonds as well. Depending on the conditions and catalysts employed, one or two molar equivalents of hydrogen will be added to a triple bond, and alkene or alkane is produced as the product, respectively.
When platinum or palladium catalysts are applied, the final product of the hydrogenation is an alkane with sufficient hydrogen provided. The initial product is an alkene, which undergoes the reaction successively to give alkane as the final product.

With a certain catalyst used, the hydrogenation of alkyne can be stopped at the alkene stage. The most commonly employed catalyst is the Lindlar catalyst. The Lindlar catalyst is prepared by precipitating palladium on calcium carbonate and then treating it with lead (II) acetate and quinoline. The special treatment modifies the surface of the palladium metal by partially deactivating it and making it more effective at catalyzing the hydrogenation to a triple bond rather than to a double bond.

The mechanism for the catalytic hydrogenation of alkyne is almost the same as that of alkene (10.5). Since both hydrogen atoms are delivered from the surface of the catalyst, they are delivered to the same side of the triple bond; therefore, syn-addition occurs. So, the hydrogenation of an internal alkyne produces cis-alkene with the Lindlar catalyst.
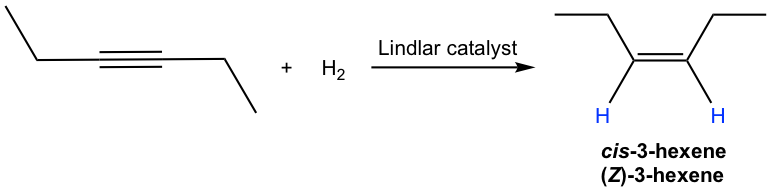
Internal alkyne can be converted into trans-alkene using sodium (or lithium) in liquid ammonia. The mechanism for this reaction involves successive single electron transfers from the metal (sodium or lithium) and proton transfers from ammonia, with radical intermediates. The sodium metal (or lithium) reacts more rapidly with triple bonds than double bonds, so the reaction stops at the alkene stage. A low temperature (-78°C) is necessary to keep ammonia in the liquid state.
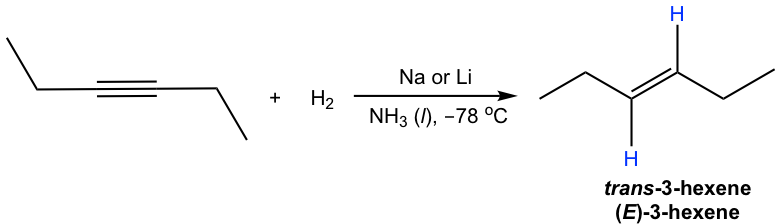
The trans-vinylic anion is formed preferentially because of the higher stability with two R groups farther apart. Protonation of the trans-vinylic anion leads to the trans-alkene.
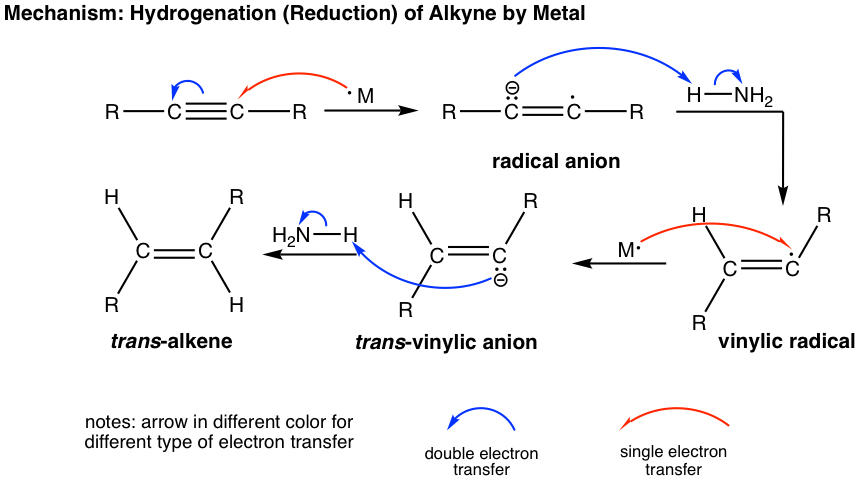
Hydrohalogenation of Alkynes
An alkyne is an electron-rich molecule with a high density of pi electrons; therefore, it is a good nucleophile that reacts readily with electrophiles. Thus, alkynes, like alkenes, also undergo electrophilic addition with hydrogen halide.
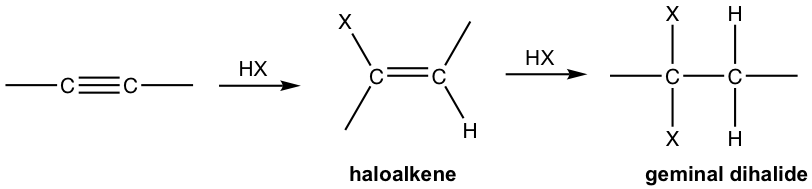
- Alkyne reacts with one mole of HX to form haloalkene and with two moles of HX to form geminal dihalides, a dihalide with both halogens attached to the same carbon. “Geminal” comes from geminus, which in Latin means “twin”.
- Both additions follow Markovnikov’s rule in terms of regioselectivity. If one molar equivalent of HX is available, the addition can be stopped at the first addition to haloalkene. The halo-substituted alkene is less reactive than alkyne for electrophilic addition because a halogen substituent withdraws electrons inductively, thereby decreasing the nucleophilicity of the double bond.
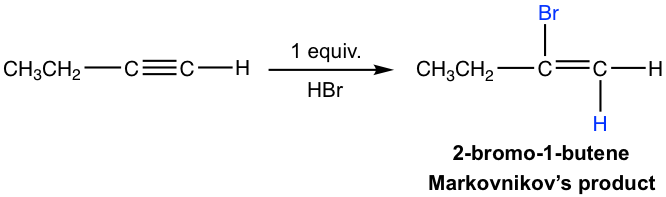
The mechanism for the electrophilic addition to alkyne is similar to the addition of alkene, with protonation as the first step. For terminal alkyne, if the protonation occurs on different triple bond carbons, the primary or secondary vinylic cation intermediate will be formed. The higher stability of the secondary vinylic cation leads to Markovnikov’s regioselectivity, in which the hydrogen atom attached to the carbon has the greater number of hydrogen atoms.

If excess hydro halide is present, the addition to alkyne occurs twice to give geminal halide that follows Markovnikov’s regioselectivity.
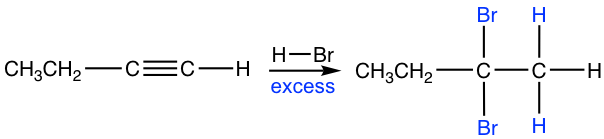
Hydration of Alkynes
Alkynes also undergo the acid-catalyzed addition of water (hydration), similar to alkenes. As a result, the H is added to one triple bond carbon and OH is added to the other triple bond carbon, and the product formed is called an enol (“en” comes from “ene” which means a double bond, and “ol” means OH group). An enol is a compound with a carbon-carbon double bond and an OH group connected to one of the double bond carbons.

Enol is a very unstable compound, and it immediately undergoes rearrangement to give a more stable carbonyl compound, either aldehyde or ketone. The structure of a carbonyl compound and an enol differ in the location of the double bond and a hydrogen atom, and they are called tautomers. The interconversion between the tautomers is called tautomerization. The mechanism is not covered. Enol always undergoes tautomerization rapidly because of the high stability of the carbonyl compound, as shown in the general way below.

For symmetrical internal alkyne that has the same group attached to each of the triple bond (sp) carbons, the addition of water forms a single ketone as a product. As in the early example, 2-butanone is produced from the hydration of 2-butyne.
For asymmetrical internal alkyne with different groups on each of the triple bond carbons, the mixture of two ketones is formed because the initial addition of the proton can occur on either of the sp carbons. The hydration of 2-pentyne is shown here, which produces a mixture of 2-pentanone and 3-pentanone as a product.
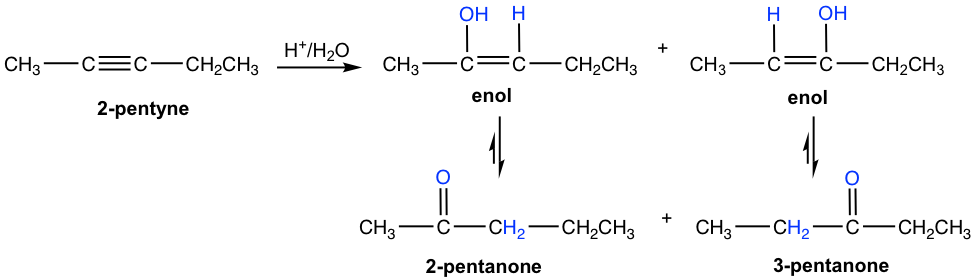
Terminal alkynes are not as reactive as internal alkynes towards hydration. The addition of water to a terminal alkyne will occur if a mercuric ion (Hg2+) is present as a catalyst. The enol formed from the addition follows Markovnikov’s rule with the hydrogen atom attached to the terminal carbon, and a methyl ketone (the ketone with a methyl group connected on one side of the C=O bond) is the final product after tautomerization.

Hydroboration-Oxidation of Alkynes:
Hydroboration-oxidation also applies to alkyne in a similar way as to alkene. The two-step process results in the enol, which goes through tautomerization to give a carbonyl compound.
Meanwhile, the addition of borane to a terminal alkyne shows the same regioselectivity as observed in borane addition to an alkene. That is, boron adds preferentially to the terminal triple bond (sp) carbon (the carbon with more hydrogen atoms), or the terminal carbon with fewer substituents. After oxidation, the boron-containing group is converted to the OH group, so the enol is produced in the anti-Markovnikov way, with OH connected to the terminal carbon. The tautomerization of such enol generates aldehyde as the final product.
Comparing the two hydration methods of alkyne, hydroboration-oxidation produces aldehyde from terminal alkyne, while acid-catalyzed hydration converts terminal alkyne to methyl ketone.
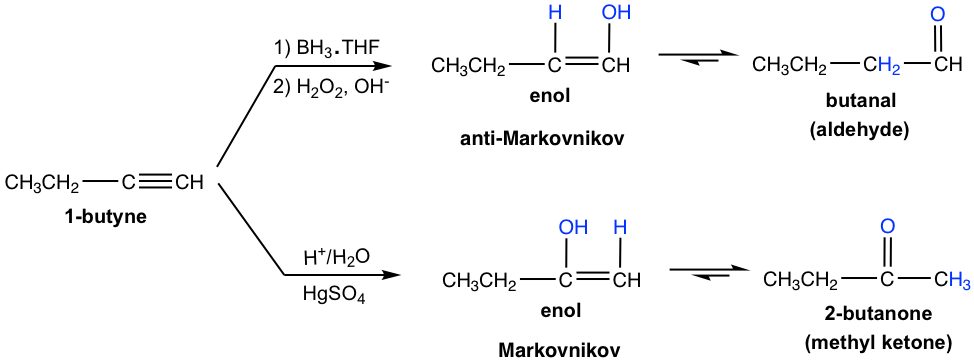