Chapter 7: Nucleophilic Substitution Reactions
7.6 Extra Topics on Nucleophilic Substitution Reactions
Our discussions so far have focused on fundamental concepts about SN1 and SN2 mechanisms, and the reactions we have learned about proceed regularly. Some other conditions can be “added” to the basic nucleophilic substitution reactions to make the reaction look different or more challenging. However, understanding the basic concepts well is very helpful for us to deal with various situations. The reaction may look different, but it is essentially still the same.
7.6.1 SN1 Reaction with Carbocation Rearrangement
Let’s take a look at an SN1 reaction.
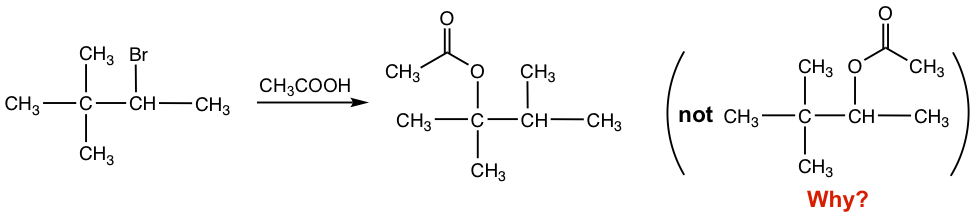
With the secondary substrate and neutral nucleophile (CH3COOH), this is an SN1 reaction, also the solvolysis that CH3COOH acts as both a solvent and nucleophile. It is supposed to give the acetate as a product, with the acetate replacing the Br. However, as shown in the reaction equation, the acetate was not introduced on the carbon with the leaving group Br but was connected to the next carbon instead. What is the reason for the unexpected structure of the product?
For reactions involving a carbocation intermediate, it is a common phenomenon that the carbocation might rearrange if such a rearrangement leads to a more stable carbocation; this is called carbonation rearrangement. Because of the carbocation rearrangement, the product of the above reaction is different than expected. This can be explained with the step-by-step mechanism below.
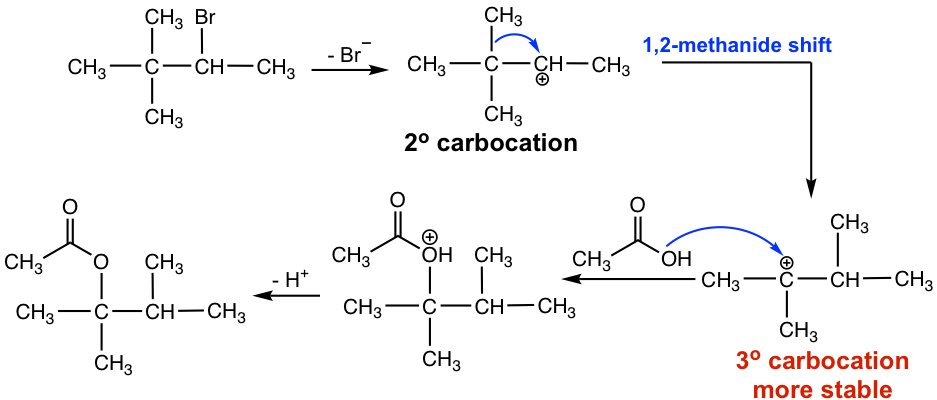
When Br– leaves, the initial carbocation formed is a secondary one. The CH3 group on the next carbon then shifts with its bonding electrons to the positively charged carbon and creates a new, more stable tertiary carbocation. The tertiary carbocation then reacts with nucleophile CH3COOH to give the final acetate product. The CH3 group shifts with the electron pair, and such a move is called 1,2-methanideshift. “1,2-“ here refers to the movement that occurs between two adjacent carbons, but not necessarily C1 and C2.
Other than the CH3 group, the H atom in other reactions could shift as well with the electron pair if such a shift can lead to a more stable carbocation. The shift of hydrogen is called a 1,2-hydride shift. A couple of notes about the carbocation rearrangement:
- Any reaction that involves a carbocation intermediate might have a rearrangement.
- Not all carbocations rearrange. Carbocations only rearrange if they become more stable as a result of the rearrangement.
- The shift is usually a 1,2-shift, which means it occurs between two adjacent carbons.
7.6.2 Intramolecular Nucleophilic Substitution Reaction
For the reactions we learned before, the substrate with a leaving group and the nucleophile are always two separate compounds. It is actually possible for one compound to contain both a leaving group and nucleophile, and the reaction occurs within the same molecule. Such a reaction is called an intramolecular (intra, Latin for “within”) reaction. A cyclic product is obtained from an intramolecular reaction.
Let’s talk about the reaction mechanism that rationalizes the structure and stereochemistry of the product for the following reaction.
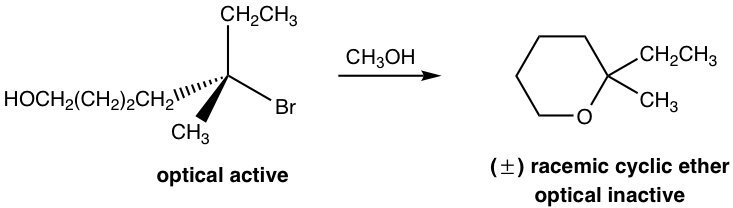
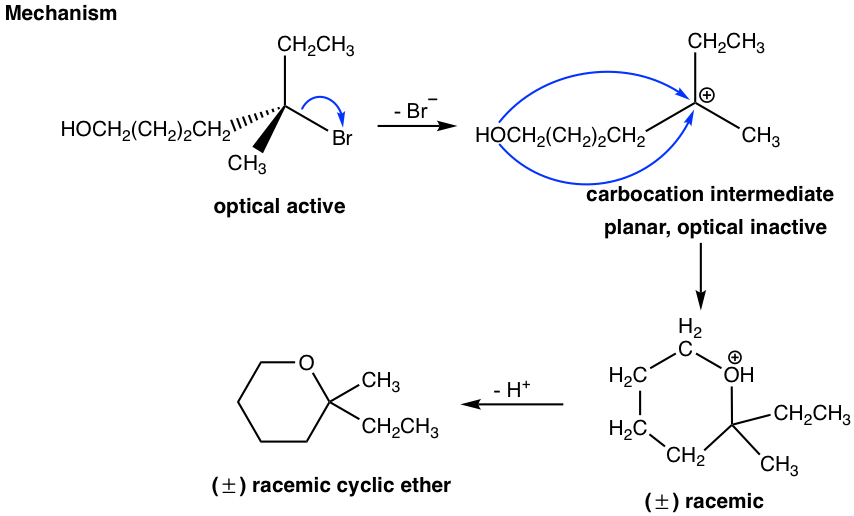
In the above reaction, the reactant has two functional groups, bromide (Br) and alcohol (OH). A compound with two functional groups is called a bifunctional molecule. In this reactant, Br is connected to a tertiary carbon, which is a good substrate for an SN1 reaction, and OH is a good nucleophile for SN1 as well, so the substitution reaction can occur within the same molecule via the SN1 mechanism. So, the reaction occurs between one end of the molecule, Br, which acts as the leaving group, and the other part of the molecule, OH, which acts as the nucleophile. As a result, a six-membered cyclic ether is formed as the product.
Since the reaction occurs with the SN1 mechanism, the carbocation intermediate is in a trigonal planar shape, and the nucleophile can attack from either side of the carbocation to give both enantiomers. Therefore, the product is a racemic mixture that is optical inactive. This is consistent with the stereochemistry feature of the SN1 reaction we learned about before.
Usually, if an intramolecular reaction can produce a five- or six-membered ring as the product, the reaction will be highly favored because of the special stability of five- or six-membered rings.
7.6.3 Converting a Poor Leaving Group to a Good Leaving Group
In early discussions about leaving groups (section 7.3), we mentioned the importance of a good leaving group for both SN1 and SN2 reactions and that the substitution reaction will not occur if a poor leaving group is present. For some situations, however, a poor leaving group could be converted to a good leaving group to make the reaction feasible. We will see a couple of strategies for such a purpose.
By Acid Catalyst H+
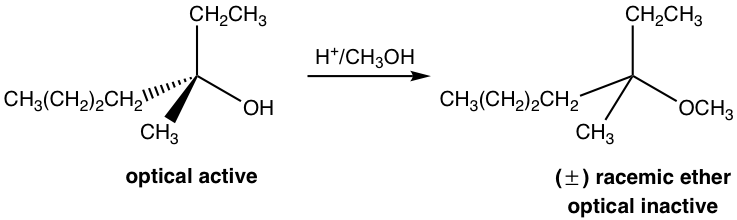
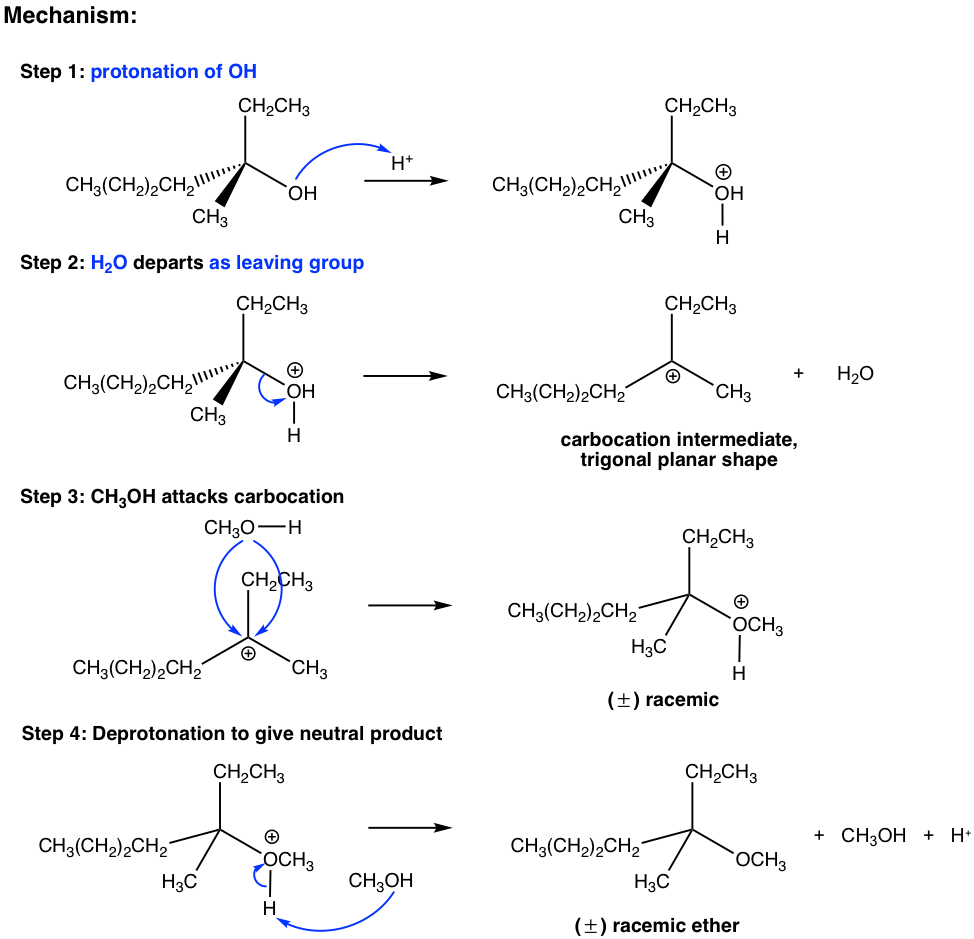
The last three steps in the above mechanism are the standard steps of the SN1 mechanism. However, the reaction will not proceed without the first step. In the first step, which is an acid-base reaction, a proton is rapidly transferred to the OH group and the alcohol gets protonated. By protonation, the OH group is converted to H2O, which is a much weaker base and therefore a good leaving group. In step 2, a water molecule departs with the electron pair and leaves behind a carbocation intermediate. The following steps are just SN1, which explains why the product is a racemic mixture. The acid H+ was regenerated in step 4 and can be reused for further reactions; therefore, only a catalytical amount of H+ is necessary to start the process.
Another commonly applied method for converting an OH group to a better leaving group is by introducing a sulfonate ester. When alcohol reacts with sulfonyl chloride, with the presence of a weak base, the sulfonate ester is formed.

As the example shown above, when p-toluenesulfonyl chloride (tosyl chloride, TsCl) is used, the resulting ester is p-toluenesulfonate (tosylate, OTs). Does the tosyl group look familiar to you? It should, as we learned about this species in section 3.2. As the conjugate base of strong acid p-toluenesulfonic acid (TsOH), OTs is a very weak base and therefore an excellent leaving group. Pyridine here acts as a weak base to neutralize the side product HCl and facilitate the reaction to completion. The detailed mechanism for this reaction is not required in this course.
Other than introducing OTs, other commonly applied sulfonyl chlorides include MsCl and TfCl, and the sulfonate ester OMs (mesylate) and OTf (triflate) are formed respectively.
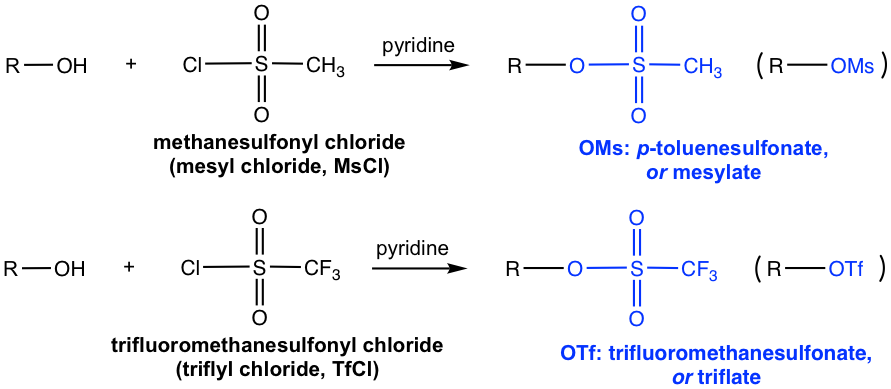
Once the primary alcohol has been converted to OTs (or OMs, OTf), it is then a good substrate for an SN2 reaction. With the appropriate nucleophile added in a separate step, for example, CH3O–, the SN2 reaction takes place readily to give ether as the final product, as shown below.
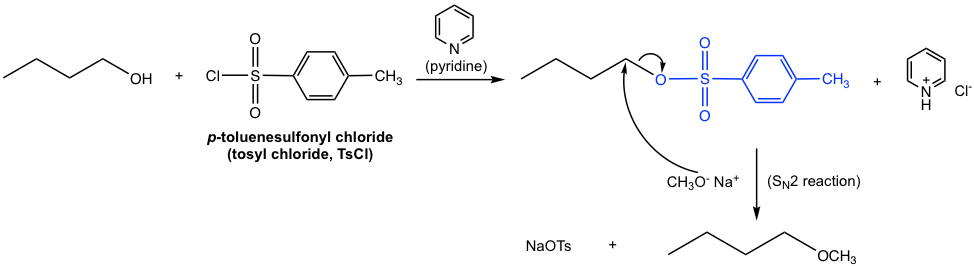
The overall synthesis of butyl methyl ether from 1-butanol involves two separate steps: the conversion of OH to OTs and then the replacement of OTs by CH3O through an SN2 reaction. The two steps have to be carried out one after the other; however, the whole synthesis scheme can also be shown below:

Note:
- Figure 7.6j represents the common and conventional way to show multiple-step synthesis in organic chemistry. The reaction conditions (reagent, catalyst, solvent, temperature, etc.) for each step are shown on top and bottom of the equation arrow. Only the structures of the starting material and final product(s) are shown, and the structures of the intermediate products for each step are not included.
- The individual steps need to be labeled as 1), 2), etc. for the proper order, and they can not be mixed together.