Chapter 10: Alkenes and Alkynes
10.1 Synthesis of Alkenes
10.1.1 Dehydrohalogenation of Alkyl Halide
The E2 elimination reaction of alkyl halide is one of the most useful methods for synthesizing alkene.
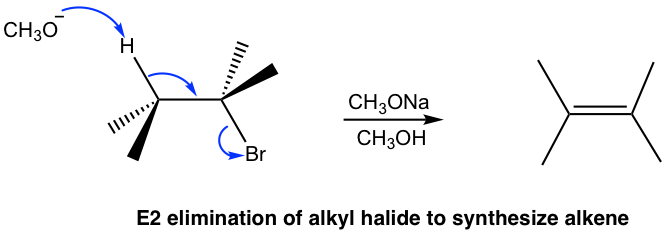
The mechanism and stereochemistry of the E2 reaction are thoroughly discussed in Chapter 8. Here are a few practical hints on making use of the E2 reaction to prepare alkene as the desired product:
- Choose a secondary or tertiary substrate if possible, since they prefer E2.
- If a primary substrate is necessary, choose a bulky base such as t-BuO– to avoid a competition of substitution reaction. As mentioned earlier (section 8.4), the primary substrate undergoes an SN2 reaction with a small species like OH–, which is also a good nucleophile.
- A high concentration of a strong base at an elevated temperature favors the E2 reaction.
- Keep in mind that a small base produces Zaitsev’s product (more substituted alkene), while a bulky base produces Hofmann’s product (less substituted alkene).
10.1.2 Dehydration of Alcohol
Other than alkyl halides, alcohols can also be the substrates for elimination to produce alkenes. Most alcohols undergo elimination by losing the OH group and an H atom from an adjacent carbon. Since a water molecule is eliminated for the overall reaction, the reaction is also called dehydration. Two dehydration reactions are shown below for synthesizing alkene from alcohol. Dehydration of an alcohol requires a strong acid with heat. Concentrated sulfuric acid (H2SO4) or phosphoric acid (H3PO4) are the most commonly used acids in the lab.
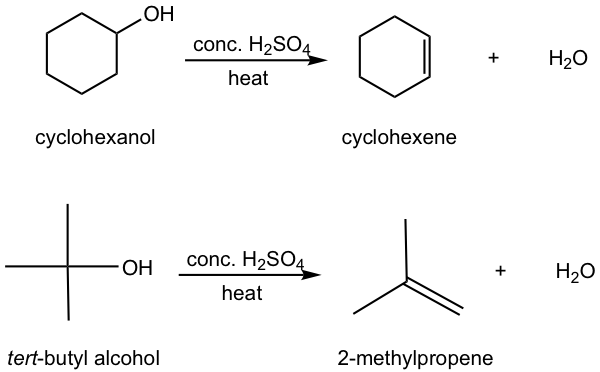
Understanding the dehydration reaction mechanism would be helpful for us to apply the method effectively. Let’s take the dehydration of tert-butyl alcohol as an example.
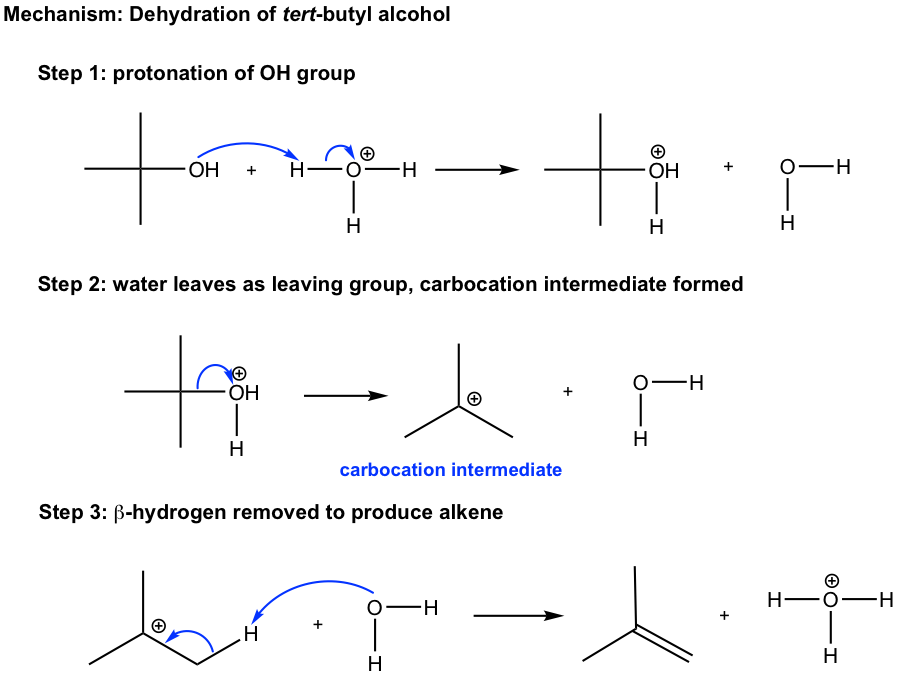
The elimination mechanism involves the carbocation intermediate, so it is essentially an E1 mechanism. However, it is not a typical E1, since it starts with the protonation step. We have learned in the substitution reaction chapter (section 7.6) that the OH group is a poor leaving group, so it never leaves. However, with the presence of strong acid (H3O+, H2SO4, etc.), the OH group is protonated by acid and therefore is converted to the good leaving group H2O. The same concepts apply here in elimination as well. Step 1 in the mechanism is the acid-base reaction for the purpose of converting the poor leaving group OH to the good leaving group H2O. Steps 2 and 3 are typical steps for an E1 mechanism. The overall dehydration reaction can be regarded as the E1 reaction of a protonated alcohol.
For the E1 mechanism, the rate-determining step is the formation of carbocation, so the relativity stability of carbocation defines the relative reactivity of alcohol towards E1 dehydration. As you can predict, the trend is:
3° alcohol > 2° alcohol > 1° alcohol (not undergoes E1 dehydration)
Another observation in the dehydration reaction is that rearrangement occurs. This makes sense because the mechanism involves the formation of carbocation. We have learned the concept in section 7.6, that a carbocation will rearrange if the rearrangement produces a more stable carbocation. An example of dehydration with rearrangement is given below:

For the dehydration of 3,3-dimethyl-2-butanol, two alkenes are obtained with 2,3-dimethyl-2-butene as the major product. However, both products have a different carbon skeleton compared to that of the reactant. This is due to the rearrangement of the carbocation intermediate, which is shown explicitly in the mechanism below.
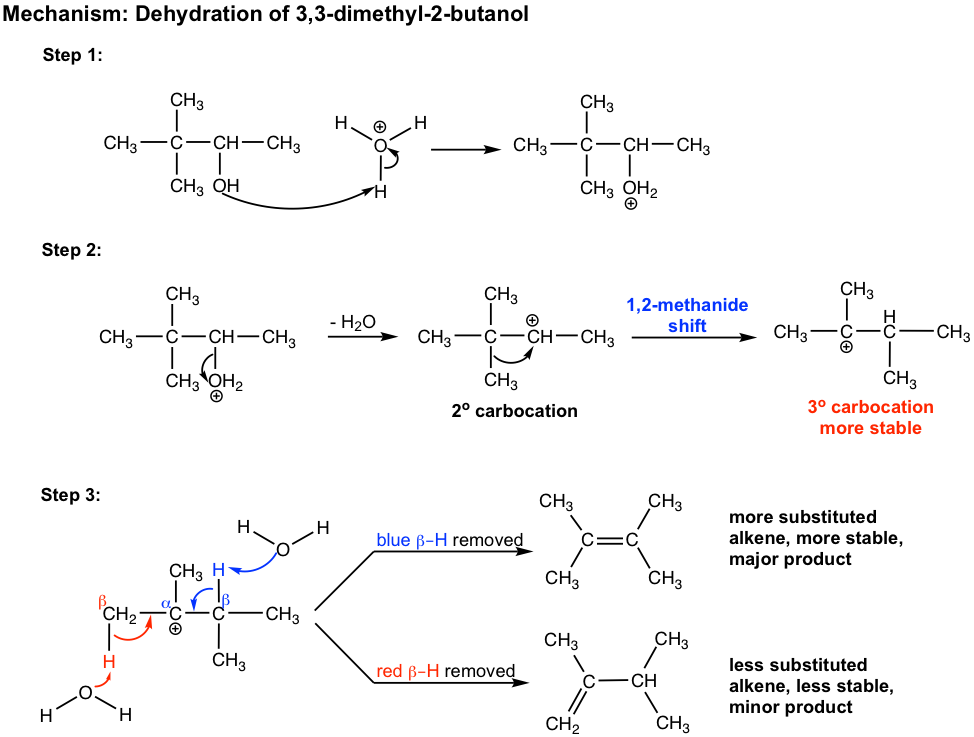
In step 2 of the mechanism, the initially formed secondary carbocation undergoes rearrangement, 1,2-methanide shift, to produce the more stable tertiary carbocation.
In step 3, there are two β-hydrogens available in the tertiary carbocation for removal. The more substituted alkene, which is more stable, is the major product.
Primary Alcohol Elimination
The primary alcohol can also undergo dehydration, however through an E2 mechanism because the primary carbocations are too unstable to be formed. The first step of the mechanism still involves the protonation of the OH group, to convert the poor leaving group to a good leaving group. The second step is the actual E2 of the protonated primary alcohol.

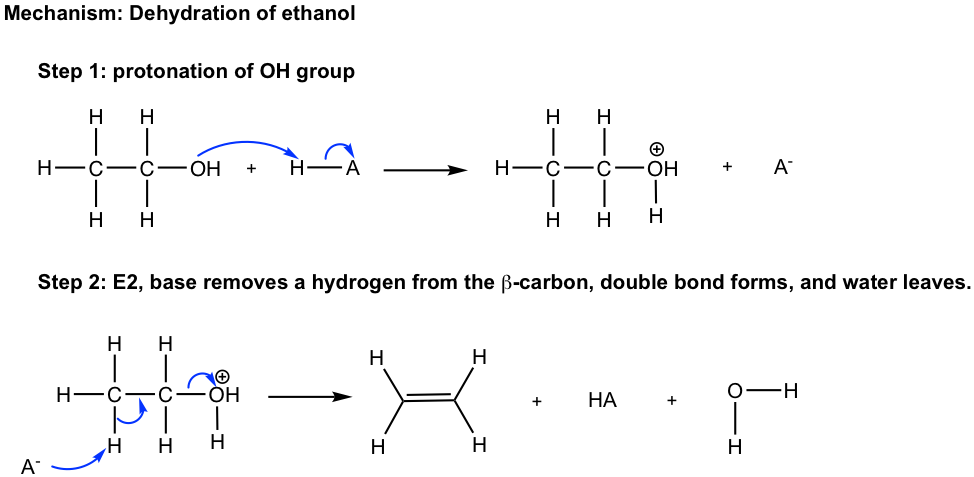