Chapter 3: Acids and Bases: Introduction to Organic Reaction Mechanism Introduction
3.4 Structural Effects on Acidity and Basicity
We have learned that different functional groups have different strengths in terms of acidity. In this section, we will gain an understanding of the fundamental reasons behind this, which is why one group is more acidic than the other. Many of the concepts we will learn here will continue to be applied throughout this course as we tackle other organic topics.
3.4.1 Element Effect
A. Periodic Trend: Electronegativity
The element effect is about the individual atom that connects with the hydrogen (keep in mind that acidity is about the ability to donate certain hydrogen). Let’s compare the acidity of hydrogens in ethane, methylamine and ethanol as shown below.
A clear trend in the acidity of these compounds is that the acidity increases for the elements from left to right along the second row of the periodic table, C to N, and then to O. This is consistent with the increasing trend of EN along the period from left to right. The connection between EN and acidity can be explained as the atom with a higher EN being better able to accommodate the negative charge of the conjugate base, thereby stabilizing the conjugate base in a better way. Therefore, the more stable the conjugate base, the weaker the conjugate base is, and the stronger the acid is. For the discussion in this section, the trend in the stability (or basicity) of the conjugate bases often helps explain the trend of the acidity.
The relative acidity of elements in the same period is:
B. Group (vertical) Trend: Size of the atom
When moving vertically within a given group on the periodic table, the trend is that acidity increases from top to bottom. This can be illustrated with the haloacids HX and halides as shown below: the acidity of HX increases from top to bottom, and the basicity of the conjugate bases X– decreases from top to bottom.
The acidity of the H in the thiol SH group is also stronger than the corresponding alcohol OH group following the same trend. For example, the pKa of CH3CH2SH is ~10, which is much more acidic than ethanol CH3CH2OH which has a pKa of ~16.
To make sense of this trend, we will once again consider the stability of the conjugate bases. When moving vertically in the same group of the periodic table, the size of the atom overrides its EN with regard to basicity. The atomic radius of iodine is approximately twice that of fluorine, so in an iodide ion, the negative charge is spread out over a significantly larger volume, so I– is more stable and less basic, making HI more acidic.
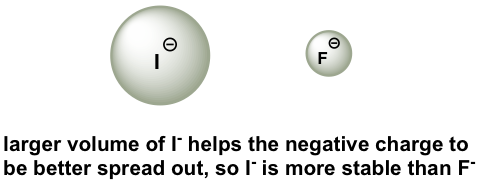
The relative acidity of elements in the same group is:
For elements in the same group, the larger the size of the atom, the stronger the acid is; the acidity increases from top to bottom along the group.
3.4.2. Resonance Effect
The resonance effect accounts for the acidity difference between ethanol and acetic acid. For both ethanol and acetic acid, the hydrogen is bonded with the oxygen atom, so there is no element effect that matters. However, the pKa values (and the acidity) of ethanol and acetic acid are very different. What makes a carboxylic acid so much more acidic than an alcohol? As stated before, we begin by considering the stability of the conjugate bases, remembering that a more stable (weaker) conjugate base corresponds to a stronger acid.
For acetate, the conjugate base of acetic acid, two resonance contributors can be drawn and therefore the negative charge can be delocalized (shared) over two oxygen atoms. However, no other resonance contributor is available in the ethoxide ion, the conjugate base of ethanol, so the negative charge is localized on the oxygen atom. As we have learned in section 1.3, the species that has more resonance contributors gains stability; therefore acetate is more stable than ethoxide and is weaker as the base, so acetic acid is a stronger acid than ethanol.
The charge delocalization by resonance has a powerful effect on the reactivity of organic molecules, enough to account for the significant difference of over 10 pKa units between ethanol and acetic acid. pKa = –log Ka, which means that there is a factor of about 1010 between the Ka values for the two molecules!
Examples
The pKa of the OH group in alcohol is about 15, however OH in phenol (OH group connected on a benzene ring) has a pKa of about 10, which is much stronger in acidity than other alcohols. Explain the difference.
Solution:
The difference can be explained by the resonance effect. There is no resonance effect on the conjugate base of ethanol, as mentioned before. However, the conjugate base of phenol is stabilized by the resonance effect with four more resonance contributors, and the negative is delocalized on the benzene ring, so the conjugate base of phenol is much more stable and is a weaker base. Therefore phenol is much more acidic than other alcohols.
Exercises 3.2
- Practice drawing the resonance structures of the conjugate base of phenol by yourself!
- It is because of the special acidity of phenol (and other aromatic alcohols), that NaOH can be used to deprotonate phenol effectively, but not to normal alcohols, like ethanol. Show the reaction equations of these reactions and explain the difference by applying the pKa values.
Answers to Chapter 3 Practice Questions
3.4.3 Inductive Effect
Let’s compare the pKa values of acetic acid and its mono-, di-, and tri-chlorinated derivatives:
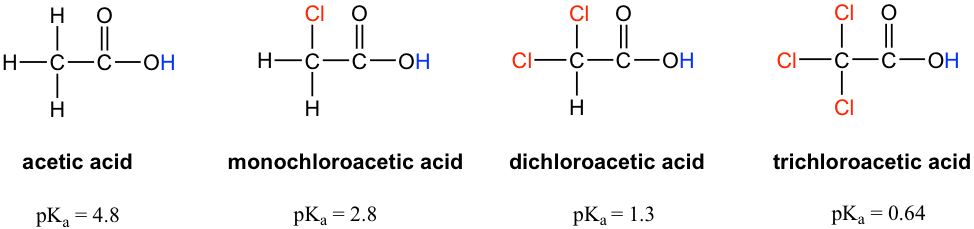
The presence of the chlorine atoms clearly increases the acidity of the carboxylic acid group, and the trend here apparently can not be explained by the element effect. The resonance effect does not apply here either, because no additional resonance contributors can be drawn for the chlorinated molecules. Rather, the explanation for this phenomenon involves something called the inductive effect. A chlorine atom is more electronegative than hydrogen and is thus able to ‘induce’ or ‘pull’ electron density towards itself via σ bonds in between, and therefore it helps spread out the electron density of the conjugate base, the carboxylate, and stabilize it. The chlorine substituent can be referred to as an electron-withdrawing group because of the inductive effect.
The inductive effect is the charge dispersal effect of electronegative atoms through σ bonds. The inductive effect is additive; more chlorine atoms have an overall stronger effect, which explains the increasing acidity from mono, to di-, to tri-chlorinated acetic acid. The following diagram shows the inductive effect of trichloro acetate as an example.
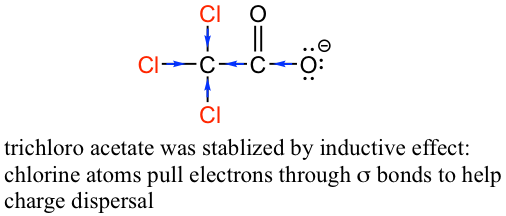
Because the inductive effect depends on EN, fluorine substituents have a stronger inductive effect than chlorine substituents, making trifluoroacetic acid (TFA) a very strong organic acid.
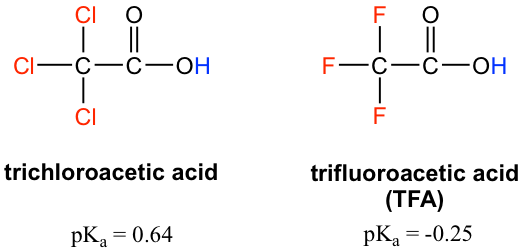
In addition, because the inductive effect takes place through covalent bonds, its influence decreases significantly with distance — thus a chlorine that is two carbons away from a carboxylic acid group has a weaker effect compared to a chlorine just one carbon away.
3.4.4 Hybridization Effect
To introduce the hybridization effect, we will take a look at the acidity difference between alkane, alkene and alkyne.
The hydrogen atom is bonded with a carbon atom in all three functional groups, so the element effect does not occur. Also, considering the conjugate base of each, there is no possible extra resonance contributor.
The key difference between the conjugate base anions is the hybridization of the carbon atom, which is sp3, sp2 and sp for alkane, alkene and alkyne, respectively. Different hybridizations lead to different s character, which is the percent of s orbitals out of the total number of orbitals. The sp3 hybridization means 25% s character (one s and three p orbitals, so s character is 1/4 = 25%), sp2 hybridization has 33.3% s character, and the number is 50% for sp hybridization. Electrons of 2s orbitals are in a lower energy level than those of 2p orbitals because 2s is much closer to the nucleus. So, for an anion with more s character, the electrons are closer to the nucleus and experience stronger attraction; therefore, the anion has lower energy and is more stable.
The relative stability of the three anions (conjugate bases) can also be illustrated by the electrostatic potential map, in which the lighter color (less red) indicates less electron density of the anion and higher stability.
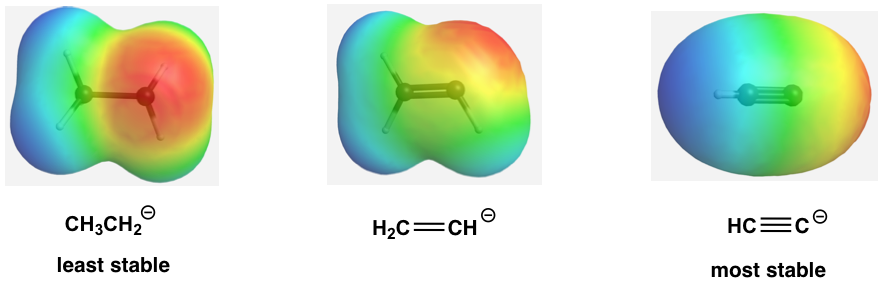
This can also be stated in a more general way as more s character in the hybrid orbitals makes the atom more electronegative. For the same atom, an sp hybridized atom is more electronegative than an sp2 hybridized atom, which is more electronegative than an sp3 hybridized atom.