Chapter 7: Nucleophilic Substitution Reactions
7.4 SN1 Reaction Mechanisms, Energy Diagram and Stereochemistry
SN1 Reaction Mechanism
The reaction between tert-butylbromide and water proceeds via the SN1 mechanism. Unlike SN2 which is a single-step reaction, SN1 reaction involves multiple steps. Reaction: (CH3)3CBr + H2O → (CH3)3COH + HBr
In step 1, the C—Br bond breaks and Br departs with the bonding electron pair to produce a tertiary carbocation and bromide anion Br–. This step only involves a highly endothermic bond-breaking process, and this is the slowest step in the whole mechanism. In the multiple-step mechanism, the overall reaction rate is determined by the slowest step, and such a step is therefore called the rate-determining step. In an SN1 reaction, step 1 is the slowest step and therefore the rate-determining step. The rate-determining step only involves the alkyl halide substrate, which is why the overall rate law is in the first order, because nucleophiles do not participate in the rate-determining step.
The product of step 1, carbocation, will be the reactant of the next step and is called the intermediate for an SN1 reaction. An intermediate is an unstable, highly reactive species with a very short lifetime. The carbocation intermediate is in a trigonal planar shape, with the empty 2p orbital particular to the plane. The central carbon is sp2 hybridized and has an incomplete octet, so carbocation is the highly reactive intermediate, which is also the electrophile.
Energy diagram of SN1 mechanism
SN1 is a multiple-step reaction so the diagram has multiple curves, and each step can be represented by one curve. Out of the three steps, the activation energy for step 1 is the highest; therefore, step 1 is the slowest step, which is the rate-determining step.
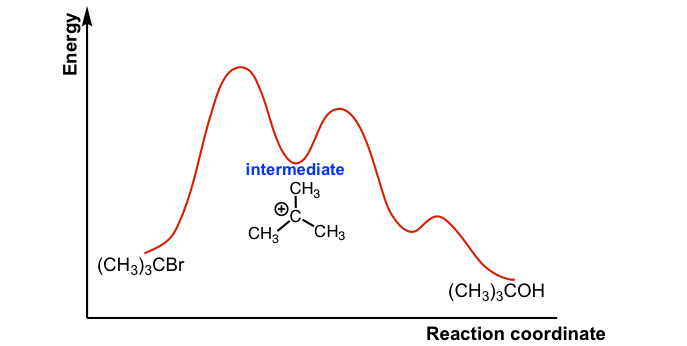
The connection between the first two curves represents the carbocation intermediate. Generally, the intermediate is the product of one step of a reaction and the reactant for the next step. The intermediate is at a relatively lower energy level compared to the transition state (which is at the peak of a curve), but the intermediate is also highly reactive and unstable.
The Effect of the Substrate Structure on the SN1 Reaction Rate
Different substrates have different reaction rates towards the SN1 reaction, and the relative reactivity of substrates towards the SN1 reaction can be summarized as:

Comparing this trend to that of SN2 reaction, you will probably realize that they are opposite. A tertiary substrate is the most reactive towards SN1, but it does not undergo SN2 at all; primary and methyl substrates are unreactive for SN1, but they are the best substrates for SN2. This comparison is very important and useful for us to choose the proper reaction condition for different substrates as we will see in the next section. For now, we will need to understand the reasoning behind the trend for SN1.
This is because of the stability of the carbocation intermediate. The mechanism shows that a carbocation is formed in the rate-determining step, so the more stable the carbocation, the more easily it is formed, and the more it facilitates the rate-determining step and speeds up the whole reaction. Therefore, the more stable the carbocation intermediate, the faster the rate of an SN1 reaction.
The relative stability of carbocation is given below, in which the tertiary carbocations are the most stable and methyl carbocation is the least stable.
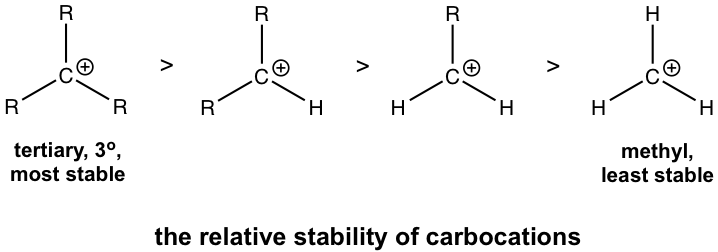
The relative stability of carbocations can be explained by the hyperconjugation effect. Hyperconjugation is the partial orbital overlap between a filled bonding orbital to an adjacent unfilled (or half-filled) orbital. The carbocation is an electron-deficient species that has an incomplete octet and empty 2p orbital. If there is an alkyl group connected with a carbocation, then there are C-C or C-H sigma bonds besides the carbocation carbon, so the filled orbitals of sigma bonds will be able to partially overlap with the empty 2p orbital. This allows sharing of the electron density with the carbocation, which stabilizes the carbocation. The more R groups involved, the stronger the hyperconjugation effect is. So, the tertiary (3°) carbocation is the most stable one. While there is no R group in the methyl carbocation, CH3+, it is the least stable.
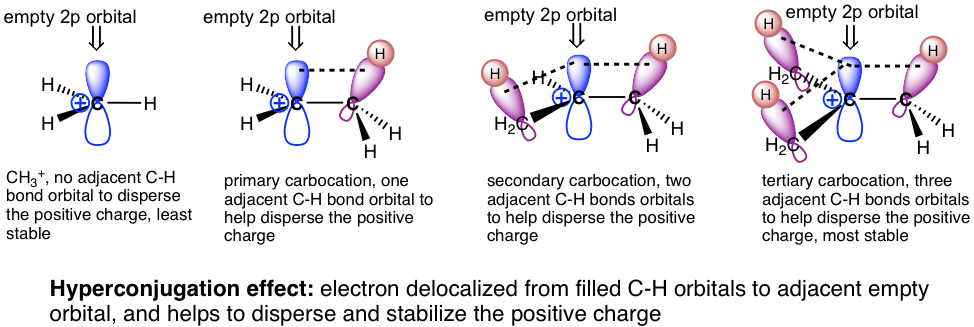
Stereochemistry of the SN1 mechanism
The stereochemistry feature of the SN1 reaction is very different from that of SN2, and of course, this can be explained well with the SN1 mechanism.
Starting with (S)-3-bromo-3-methylhexane reactant, the SN1 reaction produces a 50:50 mixture of both R and S enantiomers of 3-methyl-3-hexanol, which is the racemic mixture product. This is because the carbocation formed in the first step of an SN1 reaction has a trigonal planar shape. When it reacts with nucleophiles, it may react from either the front side or the back side, and each side gives one enantiomer. There is an equal possibility for a reaction to occur from either side, so the two enantiomers are formed with the same amount, and the product is a racemic mixture.
A reaction that converts an optically active compound into a racemic form is said to proceed with racemization. For an SN1 reaction that starts with (an optical active) one enantiomer as the reactant and the chirality center is also the electrophilic carbon (i.e. the reaction occurs on the chirality center), it proceeds with racemization as shown above.
Exercises 7.3
Show the detailed mechanism for the above reaction of (S)-3-bromo-3-methylhexane and water.
Answers to Chapter 7 Practice Questions
Please note that if the chirality center of the reactant is not the reaction center, or if there is more than one chirality center in the reactant, the SN1 reaction does not produce the racemic mixture as shown in the example below.
Examples
Show product(s) of the following SN1 reaction:
Solution:
Leaving Group Effect on SN1
As is the case for the SN2 reaction, a good leaving group is also required for the SN1 mechanism, and all the discussions we had in section 7.3 still apply.
Nucleophile
Unlike an SN2 reaction, the rate-determining step of the SN1 reaction does not include nucleophiles, so theoretically, the strength of a nucleophile does not affect the SN1 reaction. However, a strong nucleophile has a high tendency to go with the SN2 reaction instead of SN1, so a weaker nucleophile is a better choice for SN1. For the examples we have seen so far, H2O is the nucleophile.
In practice, neutral substances such as H2O, ROH, and RCOOH are usually used as nucleophiles in SN1 reactions. When these substances are applied in the reaction, they serve another function as solvents. So, they are used as both nucleophiles and solvents for the SN1 reaction, and such a reaction is also called a solvolysis reaction. A solvolysis reaction is a nucleophilic substitution in which the nucleophile is a molecule of the solvent as well. The term solvolysis comes from solvent+lysis, which means cleavage by the solvent. An SN1 reaction is usually a solvolysis reaction.
Examples
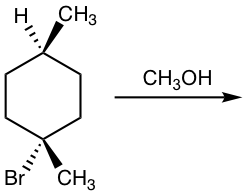
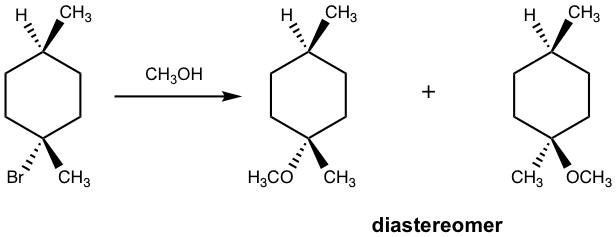