Chapter 1: Alcohols and Ethers
1.2 Reactions of Alcohols
Alcohols undergo a wide range of reactions. The understandings and discussions on the reactions of alcohol can be summarized in the following aspects:
- Acidity of the hydrogen atom of the OH group.
- Basicity and nucleophilicity of the oxygen atom of the OH group.
- Conversion of the OH group to a better leaving group (halide, tosylate, etc.) allows substitution or elimination reactions to be followed.
1.2.1 Alcohols as Acids
In the organic acids and bases section in Book I, we have explored the acidities of different functional groups, including alcohols (section 3.3 in Book I). The acidities of alcohols are generally similar to that of water, with pKa of 15 ~ 16.
Sterically hindered alcohol is usually less acidic than unhindered alcohol. For example, the pKa of tert-butanol is 18, much higher than that of ethanol which is 16. This is due to the different strengths of the solvation effect for alkoxide anions of the two alcohols. For the bulky group, t-BuO–, it is more difficult for solvent molecules to circle around, so the solvation effect is less effective than that of EtO–. As a result, the t-BuO– is more basic than EtO–, and tert-butanol is more acidic than ethanol.
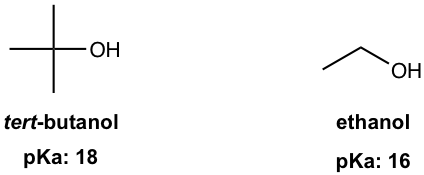
Sodium or potassium metal, and the metal hydrides (NaH or KH) are common reagents applied in organic synthesis to convert alcohols to the conjugate bases, alkoxide. Refer to the “practical tips for working on SN1 and SN2 reactions”, section 7.5 in Book I.

Alkoxides are often used in organic reactions as either strong bases or strong nucleophiles, depending on the structure of the substrate. Small-size alkoxides, such as methoxide (CH3O–) and ethoxide (CH3CH2O–), are typically good nucleophiles for SN2 when react with primary substrates. They are also strong bases for E2 reaction of tertiary substrates. The bulky alkoxide, t-BuO–, is a common choice of the bulky base that leads to Hoffmann product in E2 reaction. Refer to section 8.4 in Book I for specific examples.
1.2.2 Alcohols as Bases
The oxygen atom of the OH group is weakly basic because of the electron pairs on oxygen. With strong acid present, OH acts as a base and gets protonated.
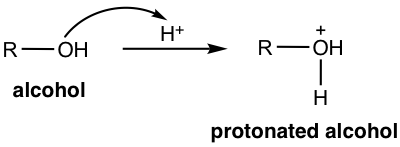
Protonation of the alcohol converts the poor leaving group OH into a good leaving group H2O, and the substrate is available for substitution or elimination reactions. The detailed reaction pathway depends on the structure of the alcohol and the reaction condition, as outlined below.
- SN2 mechanism applies to primary alcohols, when good nucleophiles present, as shown in the example below.

- Secondary and tertiary alcohols intend to react by the SN1 mechanism, in which the carbocation intermediate is involved.
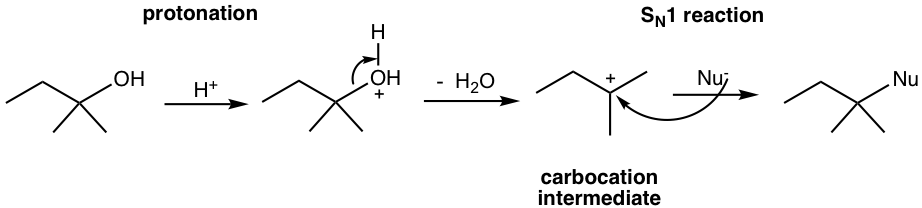
- Elimination (E1) reaction is another option for carbocation intermediate. If no strong nucleophile is present, under high-temperature reaction conditions, E1 is the major reaction pathway that produces alkene. For the following example, when tertiary alcohol is treated with concentration H2SO4, with heat, alkene is generated as the product.
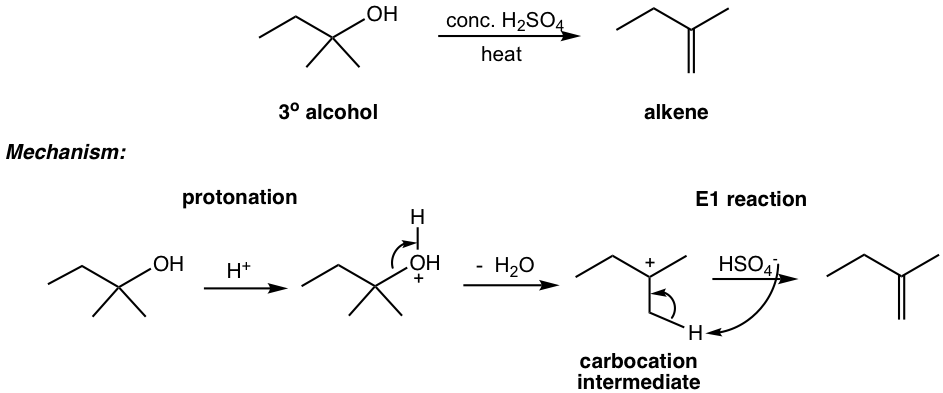
The hydrogen sulfate anion (HSO4–) present in the above reaction is a weak nucleophile and does not promote substitution reaction. Under the high temperature, the carbocation loses a proton and becomes an alkene product. You may recognize the above reaction is the dehydration of alcohol, which we learned in section 10.1.2 in Book I, a method to synthesize alkene.
1.2.3 Conversion of Alcohols into Alkyl Halides
Alcohols also undergo halogenation reactions, that is the conversion of the OH group to halides (Cl or Br). The most commonly applied reagents for such conversions are hydrogen halides (HCl, HBr), phosphorous tribromide (PBr3), and thionyl chloride (SOCl2), as shown below.
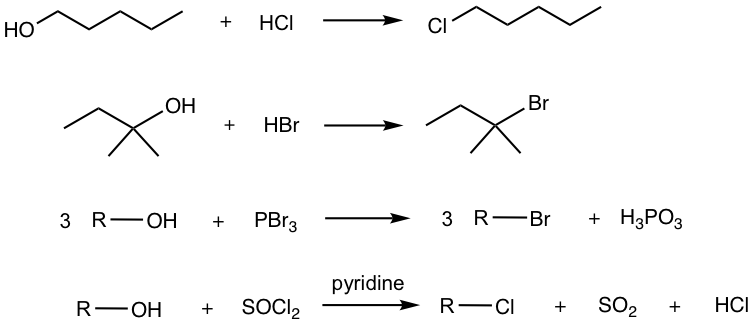
Depending on the reagents used in the reaction, the mechanism for halogenations varies a lot. The application of HX involves the multiple-step mechanisms that were just discussed in the last section 1.2.2. For strong acid HX, X– is generated after the protonation step, and acts as the strong nucleophile taking part in the following substitution step.
As mentioned in section 1.2.2 carbocation might also undergo elimination (E1) as a competitive mechanism to produce alkene (dehydration of alcohol). The competition between elimination and substitution can be controlled by applying different reaction conditions. The dehydration reaction of alcohol is usually carried in concentrated acid conditions at high temperatures; while the substitution reaction proceeds well in the presence of good nucleophile halide ions (for example Cl–) and at lower temperatures. However, it is observed often in experiments that a small amount of alkene is present as a side product in the reaction of converting alcohol to an alkyl halide.
For the reactions in which carbocation is involved in the mechanism, the possibility of rearrangement should also be considered, as illustrated in the following example.
Examples: Propose a mechanism for the following reaction.
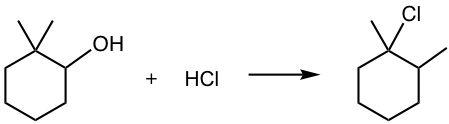
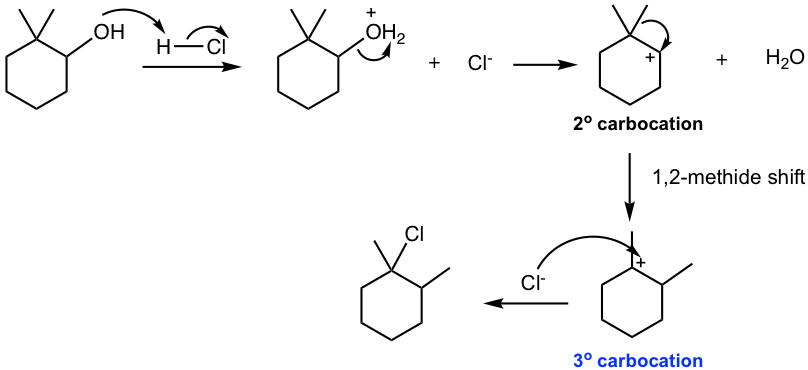
Phosphorous tribromide (PBr3) and thionyl chloride (SOCl2) are the other widely applied reagents for the conversion of alcohol to alkyl halides, please note a couple of points for the application of these reagents.

- PBr3 is often used for the transformation of alcohol to the corresponding bromide, while SOCl2 is used for the formation of chloride from alcohol.
- Both reagents work best for primary and secondary alcohols. The detailed mechanism for the reactions with these reagents will not be covered in this book, however, a note is that no carbocation is involved in the mechanism, and no rearrangements need to be concerned. This is a benefit of using PBr3 or SOCl2.
- Amine (pyridine is used most commonly) is usually needed to promote the reaction with SOCl2. A simple understanding is that amine helps to neutralize the side product HCl, thus drawing the equilibrium toward product formation. The detailed mechanism however involves more complicated steps.
1.2.4 Conversion of Alcohols into Tosylate
The reactions of alcohol with acid or hydrogen halide provide two ways of converting the poor leaving group (OH), to a better leaving group, H2O and alkyl halide respectively. Another way for such purpose is the introduction of sulfonate ester from alcohol. The most common sulfonates used are tosylates (p-toluenesulfonate esters, OTs), mesylates (methanesulfonate esters, Oms) and triflates (trifluoromethanesulfonates, OTf). When alcohol reacts with the appropriate sulfonyl chloride, with the presence of a weak base, for example, pyridine, the sulfonate ester is formed. The weak base here neutralizes the side product HCl and drives the reaction to complete.
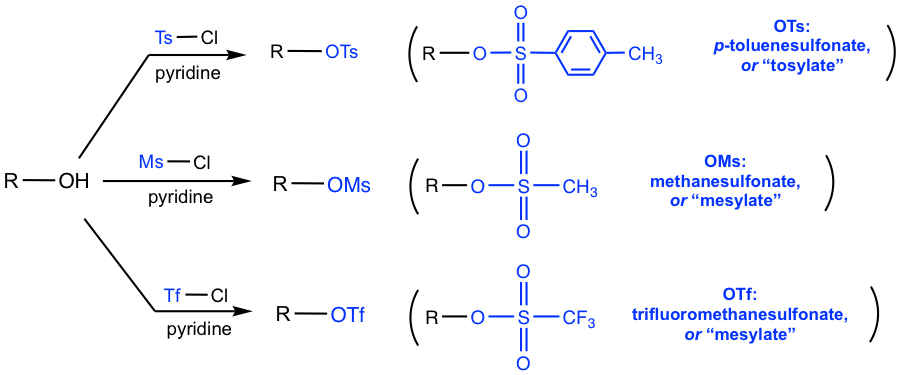
These sulfonate esters are conjugated bases of strong organic sulfonic acids (all with pKa in the negative range as shown in Fig. 1.2j), therefore they are very weak bases and as a result excellent leaving groups.
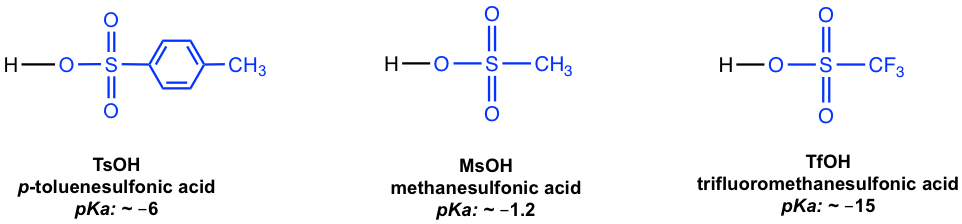
The resulting sulfonates are good substrates for substation (SN2) reactions and the overall 2-step reaction sequence provides a good synthesis strategy to convert alcohol into a variety of functional groups, for example, ether, cyanide, etc. Such applications have been discussed in the “Extra topic of nucleophilic substation reaction” in section 7.6.3 in Book I.
For alcohols with chirality centers on the carbon, introducing sulfonate ester to alcohol will not affect the stereochemistry of the chirality centers. This represents a benefit of using this strategy in the synthesis. The retention of the configuration is because the C-O bond is not involved in the formation of the sulfonate ester, therefore the stereochemistry of the alcohol carbon is not affected in this step. This strategy hence provides a way to synthesize the stereo-specific product as shown in the following example.
Example: Show the product of the following reactions with stereochemistry included.
Solution:
1.2.5 Oxidation of Alcohols
Primary and secondary alcohols are oxidized into the functional groups with higher oxidation states (refer to Fig. 10.7a in section 10.7 in Book I), when reacting with an oxidizing agent. Specifically, primary (1°) alcohols can be oxidized to aldehydes, which can be further oxidized to carboxylic acids, and secondary (2°) alcohols are oxidized to ketones. Tertiary (3°) alcohols cannot be oxidized. It also makes sense that the functional group can also be reduced from a higher oxidation state to a lower state with a reducing agent applied, and that will be covered in later section 1.4.2.
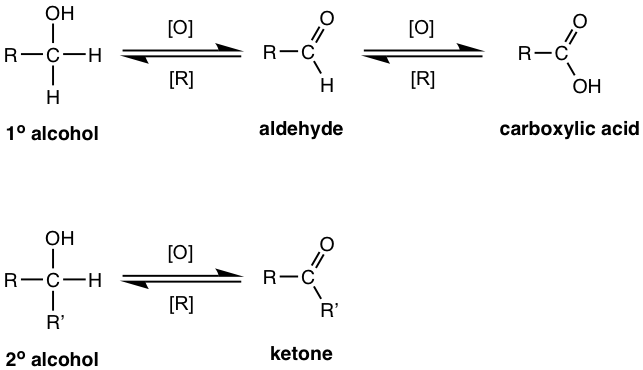
The most common oxidizing agents are the chromium (VI) reagents, such as CrO3, and H2CrO4. Such Cr (IV) reagents are also known as Jones reagents, that are prepared by adding CrO3 or Na2CrO4 to dilute sulfuric acid. The reaction can be readily handled by adding the solution of Jones reagent to aldehyde or ketone. However, the environmental hazard of Cr(IV) reagent limits its application.
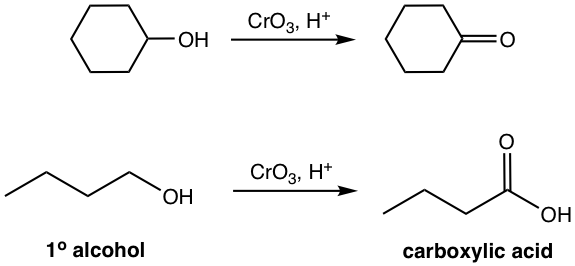
A problem with using the Cr (IV) reagent is that primary alcohols are oxidized to carboxylic directly, instead of aldehyde, due to the strong oxidizing ability of the reagent. A milder oxidizing agent, pyridinium chlorochromate (PCC), can be used instead to produce aldehyde from primary (1°) alcohol. PCC is the salt formed between CrO3, pyridine, and HCl.
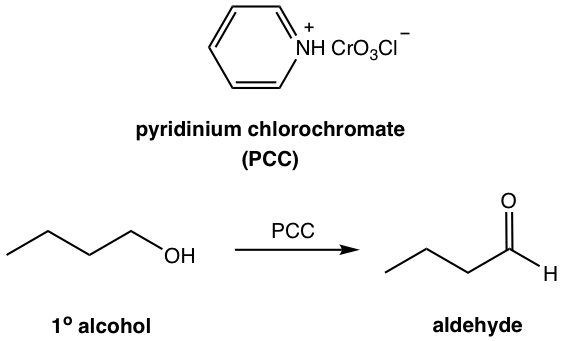
Another strong oxidizing agent, potassium permanganate (KMnO4), can also be used to oxidize primary or secondary alcohol, and produces carboxylic acid or ketone as the final product respectively. A note for using KMnO4 with primary alcohol is that since the reaction usually is carried out in a basic solution, another acidification step is necessary to produce carboxylic acid as the product.
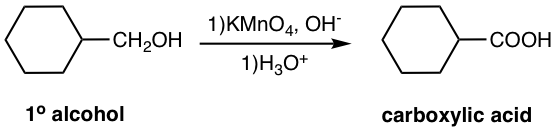