Chapter 1: Alcohols and Ethers
1.4 Synthesis of Alcohols from Carbonyl Compounds
Alcohols can also be prepared from some carbonyl compounds, i.e., the compounds containing the carbonyl group, for example, aldehyde, ketone or ester. This process involves a very important type of reaction and a group of powerful reagents, organometallic compounds, that are brand new to us.
Our journey in this topic will start with the structure features of carbonyl compounds, followed by the introduction of the new reagents, and then take a deep insight into the reactions. Other than the reactions that will be covered in this section, there will be more chapters (chapters 2, 6, 7, and 8) later to explore the properties and reactivities of carbonyl compounds.
1.4.1 Structure Features and Reactivity of Carbonyl Group Compounds
The carbonyl group is the key and common structure unit in all carbonyl compounds (refer to section 2.3 Book I).
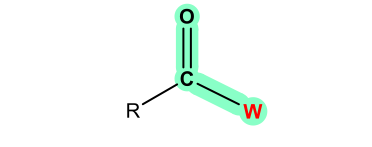
The central carbon atom in the carbonyl group is sp2 hybridized, therefore all the three atoms attached to the central carbons are in the same plane. The bond angles between any two bonds are about 120 and the overall shape can be described as trigonal planar.
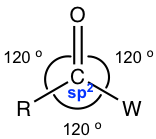
The C=O bond is highly polarized because of the electronegativity difference between the carbon atom and the oxygen atom. The electron density is attracted strongly to the O side because of the high electronegativity of the oxygen atom. Therefore, the oxygen atom bears a substantial negative charge and the carbon atom bears a substantial positive charge, which can be represented by the following structures.
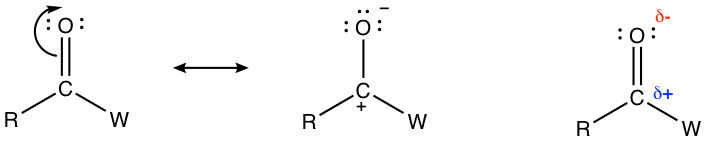
Because of the strong polarity of the C=O bond, carbonyl compounds usually exhibit a large dipole moment value. The example of acetone is shown in Fig. 1.4d.
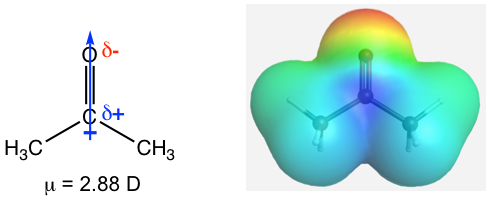
The partial charge separation on the atoms of the carbonyl group plays a critical role in the reactivity of carbonyl compounds.
Because of the partial positive charge on the carbon atom, the carbonyl group is highly susceptible to nucleophilic attack (Fig. 1.4e). For aldehydes and ketones, the carbonyl compounds we will focus on in this section, nucleophilic addition is one of the most important reactions.
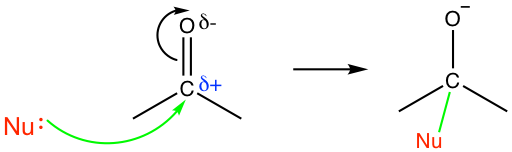
In the above nucleophilic attack, the electron pair (negative charge) of the nucleophile is attracted by the partial positive charge on the carbon atom, and a new bond is formed when the nucleophile adds to the carbonyl carbon. As a result, the electron pair in the C=O double bond shifts to the oxygen atom to generate an oxide anion. With the reaction taking place, the structure of the carbon atom changes from sp2 hybridization with a trigonal planar shape to sp3 hybridization with a tetrahedral shape.
The simple idea of positive charged (or partially positive charged) species attracted by and reacts with negative charged (or partially positive charged) species is the underlying theory for understanding a lot of different reactions. Keeping this idea in mind is very useful! The nucleophilic addition here is a typical example of this theory, and so are the SN1/SN2 reactions we learned in Book I.
Now we understand that the carbonyl carbon is ready to react with nucleophiles, in the following sections we will introduce a couple of metal reagents, metal hydrides and organometallic reagents, the new reagents that act as nucleophiles.
1.4.2 Reduction of Carbonyl Compound by Metal Hydrides
For metal hydrides, the hydride ion (H–) acts as a nucleophile to attack the carbonyl carbon. The most commonly applied metal hydrides are lithium aluminum hydride (LAH, with the formula of LiAlH4) and sodium borohydride (NaBH4) with structures given here.
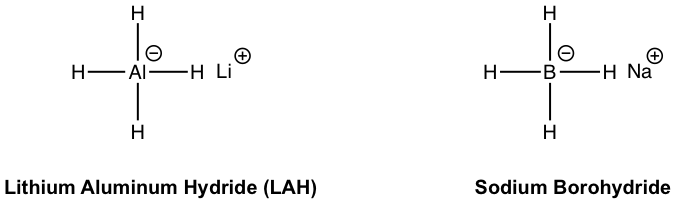
The common feature of these two reagents is that they both have hydride anion (H–) that acts as a nucleophile to react with the carbonyl group. However, they also show different reactivities. Lithium aluminum hydride is a very strong reducing agent that can reduce most carbonyl compounds including carboxylic acid, ester, aldehyde, and ketone. Sodium borohydride could only be used to reduce aldehyde and ketone because of its lower reactivity.
On the other hand, the reaction conditions required for handling the two reagents are also different due to the reactivity difference. As a side effect of the high reactivity, LAH also reacts violently with protic solvents that contain protons, such as water and alcohol. Anhydrous diethyl ether (Et2O) is therefore the solvent that is commonly used for LAH reduction, with great care being given to avoid the presence of any moisture, as shown in the general reactions in Fig. 1.4g.
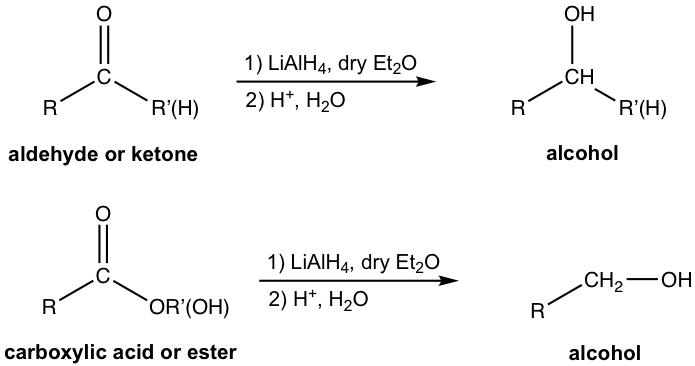
When all of the LAH has been consumed, dilute acid is added to neutralize the salt. The last step is usually called the “acidic work-up” step. Please note that the two steps must shown as step 1) and step 2) separately in proper order, which means that after the reaction with LAH is completed in step 1), acid will be added as the second step to finish the whole process.
Sodium borohydride, on the other side, is a less powerful reducing agent and can be used safely with alcohol as the solvent.
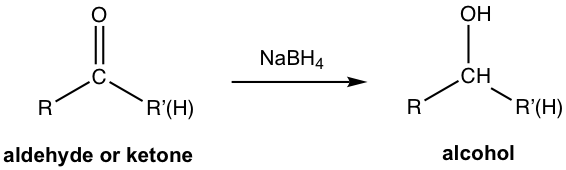
The above reactions represent effective ways to synthesize alcohol. Because alcohols are in a lower oxidation state than carbonyl compounds, they are reduction reactions. The reduction of aldehyde produces primary alcohol, the reduction of ketone produces secondary alcohol, and the reduction of acid/ester by LiAlH4 produces primary alcohol only.
The mechanism for the reduction of aldehyde/ketone by NaBH4 is shown in Fig. 1.4i. The overall mechanism is an addition to the carbonyl group, which represents a good example of the reactivity of the carbonyl group shown in Fig.1.4e. Hydride ion (H–) first acts as a nucleophile to attack the carbonyl carbon that bears a partial positive charge, the resulting oxide is protonated by the solvent to give alcohol as the final product. Such a reaction is also called a nucleophilic addition reaction.
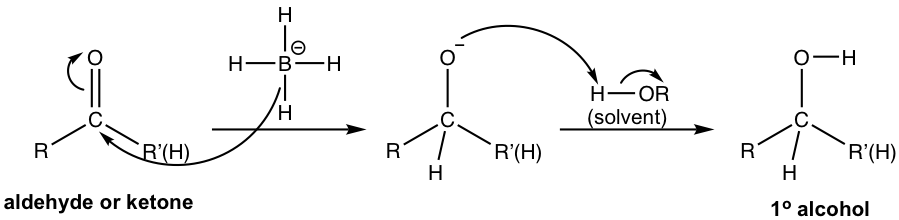
LiAlH4 reduces aldehyde/ketone by the same mechanism. The mechanism for the reduction of carboxylic acid/ester by LiAlH4 will be discussed in Chapter 7.
1.4.3 Organometallic Compounds
Another type of reagent that acts as a nucleophile is called the organometallic compound.
Carbon element is the key element in all organic compounds. In the organic structures we have learned so far, covalent bonds are always formed between the carbon element and other non-metal elements, such as carbon, hydrogen, oxygen, sulfur, phosphorous elements, etc. The carbon element can also bond with metal elements, and that is the key feature of this new type of compound: organometallic compounds.
An organometallic compound is a type of compound that contains carbon-metal bonds.
Because metals are usually less electronegative than carbon, the carbon-metal bond is polar with the carbon element bearing the partial negative charge, and the metal element bearing the partial positive charge. (pg 556)
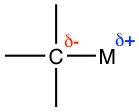
We will focus on the properties and applications of the two most common organometallic compounds: organolithium and organomagnesium compounds in this section.
Organolithium Compounds
The general way of preparing organolithium compounds is by reacting lithium metal with alkyl halide. These reactions are usually carried out in dry ether solvent (Et2O or THF) to avoid the side reaction between moisture with the resulting organolithium compounds.
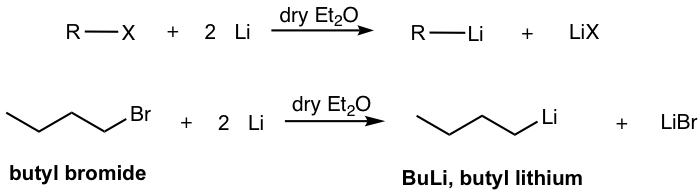
The name of organolithium compounds starts with the name of the alkyl group, followed by “lithium”. In the above example in Fig. 1.4k, the compound with formula BuLi is named butyl lithium.
Organomagnesium Compounds (Grignard Reagents)
The reaction between organic (alkyl or aryl) halide and magnesium metal in dry ether solvent generates organomagnesium compounds, which are commonly called Grignard Reagents. The reagents were first discovered by French chemist Victor Grignard in 1900. Grignard received the Nobel Prize in 1912 for this great discovery that has important applications in organic synthesis.
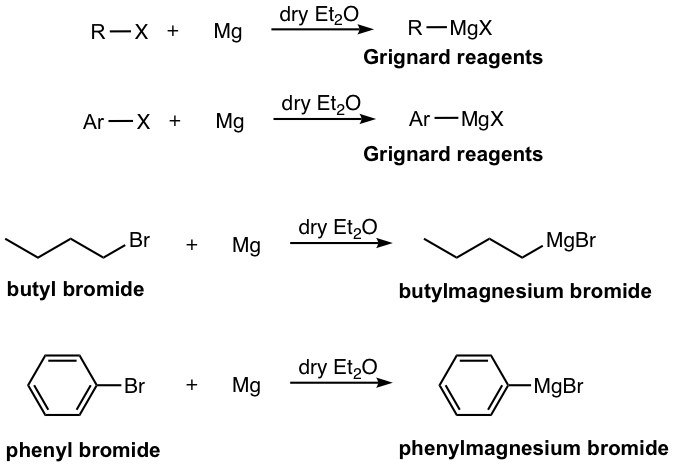
Grignard reagents can be prepared from both alkyl halide and aryl halide (as shown in Fig. 1.4l), which covers a broader structure scope than lithium reagents. The order of reactivity for halides is RI > RBr > RCl. Alkyl or aryl bromides are used most commonly because of the median level reactivity. Grignard reagents are named “alkyl”+”magnesium”+“halide”.
The mechanism for the formation of Grignard reagents is complicated and debatable, with the possible involvement of radicals. Although the actual structures could be more complex, the general formula of Grignard reagent is usually represented in a simplified way as RMgX, with Mg inserts between the carbon and the halogen.
1.4.4 Reactions of Organometallic Compounds with Carbonyl Compounds (for Alcohol Preparation)
The reactivities of these two organometallic reagents can be more easily understood if they are regarded as the structures involving carbanion and the metal cation, although the actual bonds are not completely ionic.
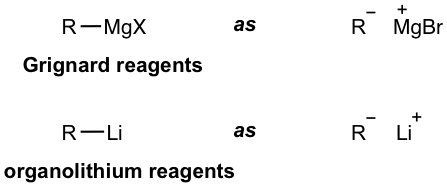
As discussed earlier (Fig. 1.4e) the carbon atom in the carbonyl group is highly susceptible to nucleophilic attack. The carbanions in these two organometallic compounds, with electron pair and negative charge, are strong nucleophiles that are ready to attack the carbonyl carbon. As a result, the most important synthetic applications of these two organometallic compounds are those in which they react as nucleophiles and attack the carbonyl group.
Grignard Reactions
Let’s start with the Grignard reagents. The reaction and mechanism of Grignard reagent with carbonyl compound are shown in Fig. 1.4n. Two steps are involved in the general mechanism. First, the carbonation (R–) of the reagent acts as a nucleophile to attack the carbonyl carbon, a new bond is formed and the π electron pair of the carbonyl group moves to the oxygen atom to form an intermediate oxide with a tetrahedral shape. In the second step (the work-up step), the oxide is protonated with acid to produce the neutral alcohol product, together with MgX2 (the inorganic side product). Similar to the reaction conditions for LiAlH4, the two steps must be shown separately with proper order on the overall reaction arrow (Fig. 1.4n).
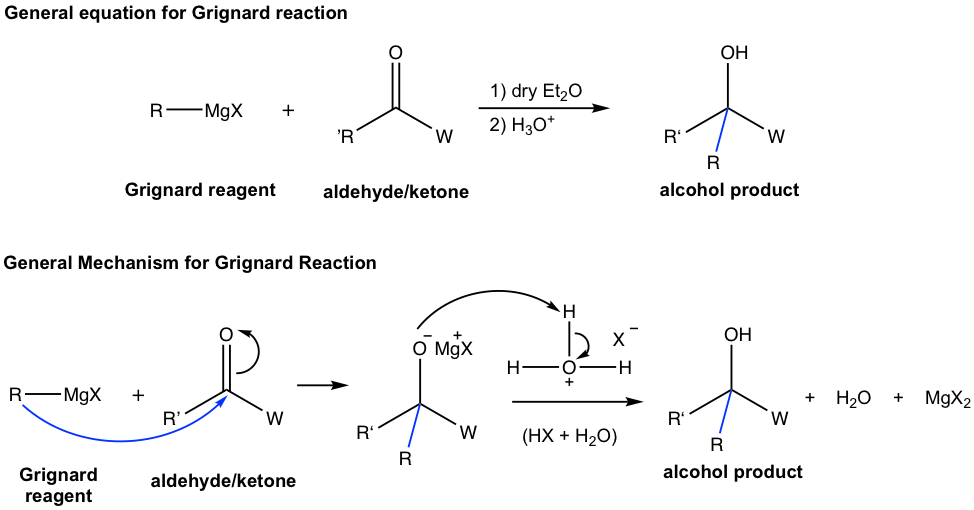
Generally speaking, alcohols are obtained as products of the reaction between Grignard reagents and carbonyl compounds. Depending on the structure of specific carbonyl compounds employed, alcohols with different categories (1º, 2º, or 3º) are formed as summarized in Table 1.3 explicitly. Please note that the Grignard reagent reacts with ester as well, to produce the 3º-alcohol. Since ester reacts with two equivalents of Grignard reagents, there are two identical alkyl groups present in the 3º-alcohol both come from the Grignard reagent.
Carbonyl Compound | Product | Specific Example |
Formaldehyde | 1° (primary) alcohol | ![]() |
Other aldehydes | 2° (secondary) alcohol | ![]() |
Ketones | 3° (tertiary) alcohol | ![]() |
Esters | 3° (tertiary) alcohol with two identical substituents | ![]() The mechanism for Grignard reaction with ester does not fit the mechanism in Fig. 1.4n. It will be covered in detail in section 7.8.2. |
Table 1.3: Alcohol Products by Grignard Reaction of Different Carbonyl Compounds
Special Note: Grignard Reaction of Carbon Dioxide CO2
Carbon dioxide is not a carbonyl compound. However, the C=O double bond in CO2 does undergo the Grignard reaction in a similar way as other carbonyl compounds and produces carboxylic acid as the product. This is a useful method for the preparation of acids (more discussions about acids in chapter 7).
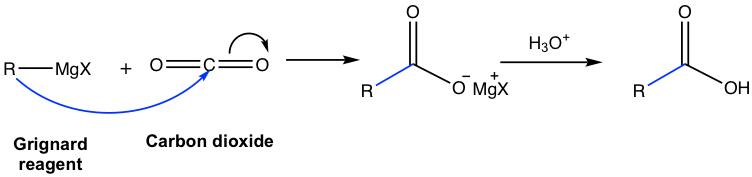
Grignard reactions provide opportunities to build larger molecules, this is exactly what chemists are working for in organic synthesis, to synthesize more complicated molecules with more carbons. As a result, the Grignard reaction is a very useful reaction and has been applied broadly in organic synthesis.
Organolithium Reactions
Organolithium reagents (RLi) react with aldehyde/ketone in the same way as Grignard reagents and provide an alternative method for alcohol preparation. While organolithium reagents are more reactive, they are more difficult to handle, and more strict dry reaction conditions are required.
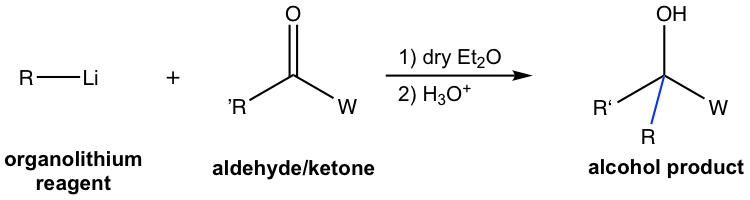
1.4.5 Synthesis Design of Grignard Reactions for Preparation of Alcohols
The diversity and good compatibility of the Grignard reaction allow us to synthesize alcohol with almost any structure. To design the synthesis, we need to choose the proper Grignard reagent and the proper carbonyl compound by examining the structure of the desired alcohol, and applying the retrosynthesis analysis strategy (section 9.6 in Book I). There might be more than one reasonable way to achieve the target in many cases.
Before we start the synthesis route designing, let’s first examine a Grignard reaction (the 3rd example, the synthesis of 3-methyl-3-hexanol, in Table 1.3) in more detail and obtain an in-depth understanding of what’s going on in the reaction.
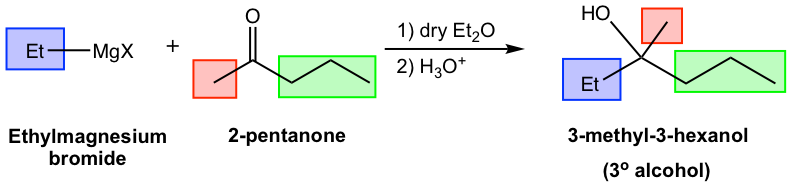
For the above example (Fig. 1.4q), the alkyl groups in both reactants and product are highlighted with different colors for your reference. In the tertiary (3°) alcohol product, the red and green groups come from ketone, and the blue group comes from the Grignard reagent. Such a trend can be further visualized in the general equation of the Grignard reaction below in Fig. 1.4r.
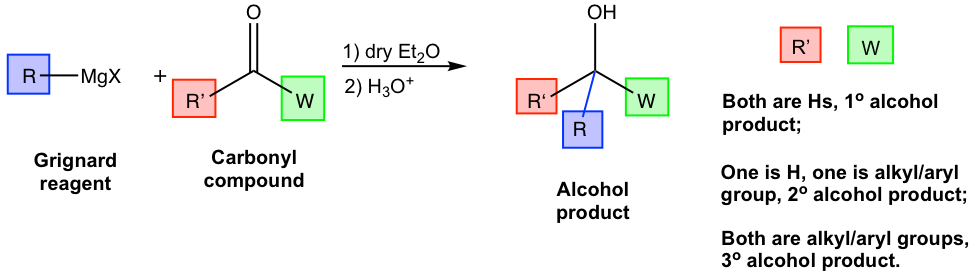
For the three groups bond on the alcohol carbon in the product, one (blue) comes from the Grignard reagent, and the other two (red and green) come from the carbonyl compounds. Depending on the two (red and green) groups from the carbonyl compounds are either both hydrogens, one hydrogen and one alkyl/aryl group, or both alkyl/aryl groups, the resulting product can be 1°, 2°, or 3° alcohol respectively.
This is a very important and useful trend that can be applied in synthesis design. By analyzing the three groups (or hydrogens) involved in the alcohol product, we can decide what reactants to start with. Theoretically speaking, there is no restriction about which groups come from carbonyl compounds or Grignard reagents, and there are usually multiple ways to synthesize a product. However, if any restrictions/requirements are given in a question, that limits your choice to one possible way. Let’s take a look at a couple of examples below for illustration.
Example A:
Design a Grignard synthesis of the following compound:
Analysis:
Step 1: This is a 2° alcohol, so one of the three groups bond on the alcohol carbon is hydrogen.
Step 2: Decide the other two groups connected to the alcohol carbon by cutting the target compound into multiple pieces. So one group is methyl (CH3), and the other group is phenyl.
Step 3: Now we can decide the structure of the reactants. While there is no other restriction for the reactants for this question, there are two options available.
Option 1: Phenylmeganesium bromide and acetaldehyde
Option 2: Methylmeganesium bromide and benzaldehyde
Note: Please double check that the number of carbons in the product equals the add-up of the number of carbons in both reactants, to make sure you did not miss out or use too many carbons.
Answer:
Synthesis method I:
Synthesis method II:
Example B:
Outline a synthesis of the following compound by using halides and carbonyl compounds of no more than four carbon atoms as organic starting materials. Other inorganic compounds and solvents can be used as necessary.
Analysis:
By applying the strategy we have learned, there are three options available for combinations of Grignard reagents and ketones for this question, as shown here. However, because of the extra requirement that all organic reagents should have no more than four carbons, only option III works as the solution to this question.
Please note that such extra requirements are very common for synthesis design questions.
Answer: