Chapter 9: Carbohydrates
9.2 The Cyclic Structure of Monosaccharide and Mutarotation
The structure of monosaccharides has two categories: the open-chain form and the cyclic form. The discussions in previous sections focused on the open-chain form, and we will start with D-glucose as an example here to illustrate the cyclic form structures, and the correlation between the two types of structures.
9.2.1 The Cyclic Structures of Monosaccharide
The reason why D-glucose exists in cyclic form is that the molecule contains both aldehyde and alcohol functional groups. The intramolecular reaction between the aldehyde group (on C-1) and the OH group on C-5 forms the six-membered cyclic hemiacetal functional group (refer to section 2.5 for the formation of hemiacetal).
The detailed process of converting from open-chain D-glucose in Fisher projection to cyclic form is shown in Fig. 9.2a diagram.
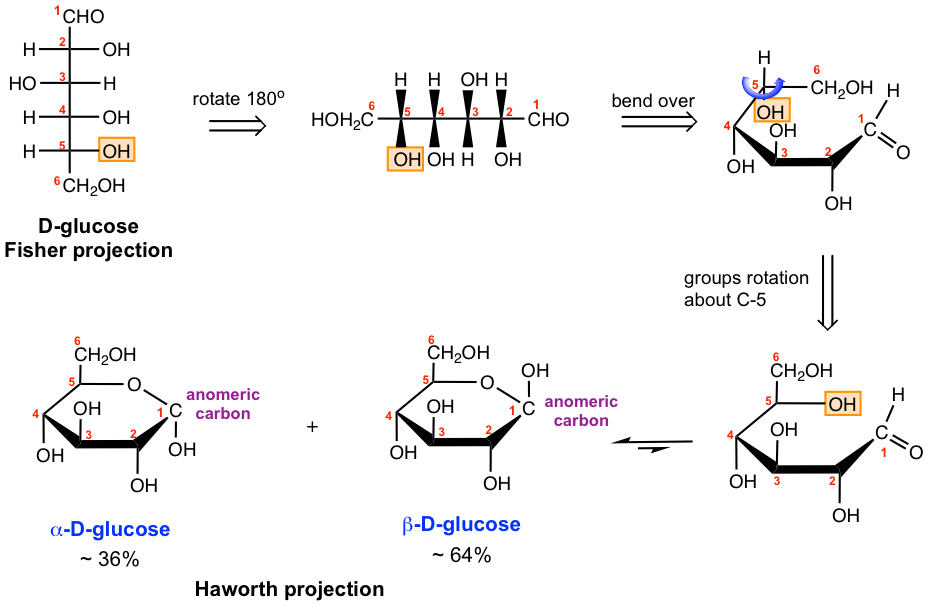
To make it easier for us to see the correlation between open-chain and cyclic structure, the Fisher projection is first rotated 90° and re-drawn horizontally (please note that this drawing is not a Fisher projection anymore). The structure is then bent over to mimic a flat ring structure, with the groups on the right side in the Fisher projection point down in the “ring”, and the groups on the left side in the Fisher projection point up in the “ring”. Next, the groups around C-5 are rotated so that the CH2OH group points “up” and the OH group stays “flat”, as a result, the OH group is in a “convenient” position to react with the aldehyde group on C-1 and forms the six-membered hemiacetal ring. The structure then could be easily converted to the ring structure. With the formation of the hemiacetal group, C-1 is a newly generated chirality center in the ring that could have two possible configurations. Therefore two diastereomers exist for the cyclic hemiacetal and they are called α-D-glucose (OH group points down of the ring, and trans to the CH2OH group) and β-D-glucose (OH group points up of the ring, and cis to the CH2OH group) respectively. In carbohydrate chemistry, the diastereomers differing only at the hemiacetal (or acetal) carbon are called anomers, and the hemiacetal (or acetal) carbon is called the anomeric carbon. α-D-glucose and β-D-glucose are two anomers of D-glucose. The prefixes α and β are always used to denote the configuration of the anomers in carbohydrate chemistry.
The way of six-membered ring hemiacetal shown above is called the Haworth projection.
Studies of the structure of the cyclic hemiacetal of the D-glucose have demonstrated that the actual conformation of the rings is the chair conformation similar to cyclohexane. Almost all the large substituents, OH and CH2OH, are equatorial in the chair conformations of D-glucose, except on the anomeric carbon for α anomer, as you would expect. In carbohydrate chemistry, both Haworth projections and chair conformations are commonly used to represent the structures of the cyclic form sugars.
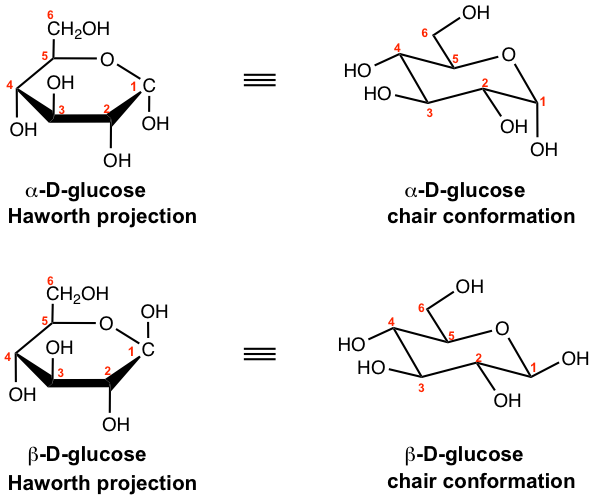
Other than six-membered rings, five-membered rings are also common for cyclic forms of monosaccharides. D-ribose, the pentose, for example, forms the five-membered-ring hemiacetal, again in both α and β anomers.
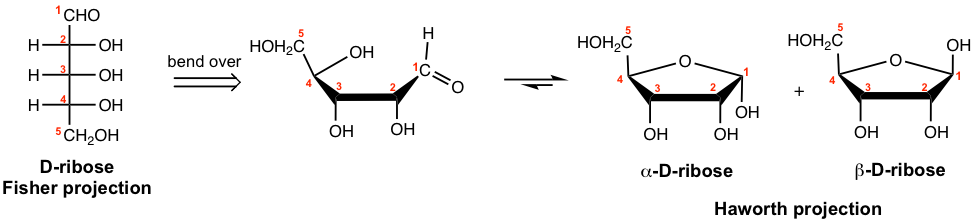
A six-membered cyclic monosaccharide is called a pyranose, and a six-membered cyclic monosaccharide is called a furanose. These names come from the names of heterocyclic compounds pyran and furan, as shown in Fig. 9.2d.
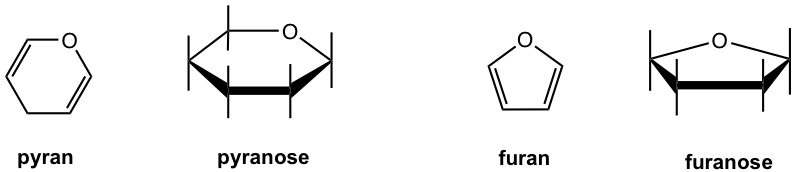
Thus, the full name of the β-D-glucose, for example, is β-D-glucopyranose. A couple more examples of cyclic monosaccharides are given below with the full names.

9.2.2 Mutarotation
As we have learned D-glucose exists in three forms, the open-chain form and two cyclic forms (α and β anomers). Further studies indicated that pure α-D-glucopyranose has a specific rotation of +112°, and β-D-glucopyranose has a specific rotation of +18.7°. However, when crystals of pure α-D-glucopyranose are dissolved in water, the optical rotation of the solution changes gradually from +112° to +52.7°. Similar observations apply to the solution of pure β-D-glucopyranose as well, with the optical rotation of the solution changing gradually from +18.7° to +52.7°. This slow change in optical rotation toward an equilibrium value is called mutarotation.
The explanation for the mutarotation lies in the existence of an equilibrium between the open-chain form and the α and β forms of the cyclic hemiacetals in water, as shown below with the cyclic forms in both Haworth projections and chair conformations for your comparison.
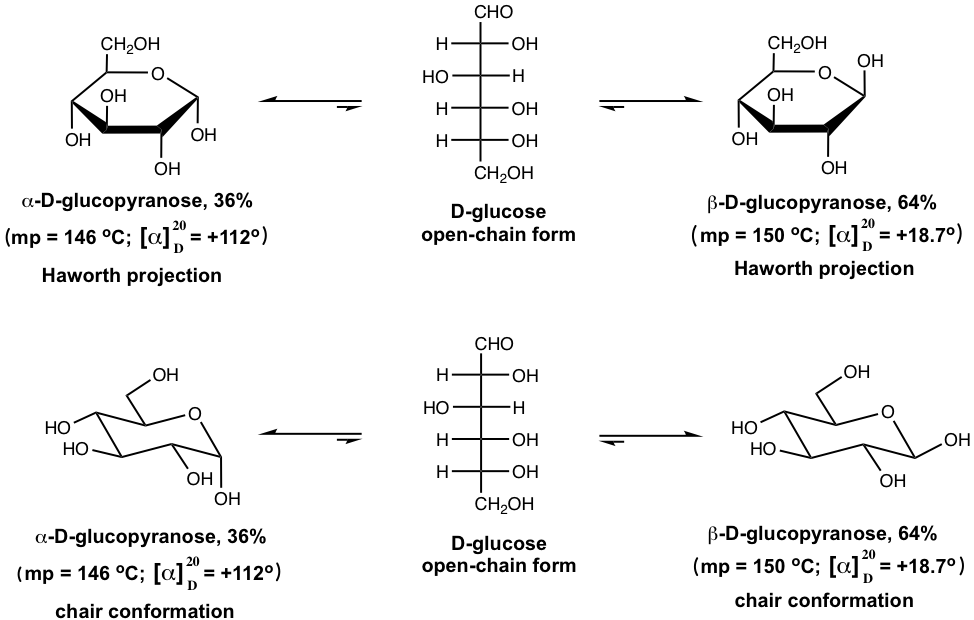
In solution, the hemiacetal ring opens to form the open-chain form, and the open-chain form recyclizes to form cyclic hemiacetals, till an equilibrium is reached with a certain concentration/amount of each form present. The concentration of open-chain form in solution at equilibrium is very small for D-glucose, which is about 0.02%. This is supported by the evidence that the IR of the solution of D-glucose has no band for a carbonyl group. Assuming that the amount of open-chain form is negligible, the percentages of each cyclic form can be calculated based on the specific rotation for each (+112° for α, and +18.7° for β), and the observed rotation for the solution at equilibrium (+52.7°). The higher percentage of β anomer, which is 64%, can be explained by the higher stability of β-D-glucopyranose with all the groups in equatorial positions.
9.2.3 Glycoside Formation
When D-glucopyranose reacts with alcohol under acidic conditions, the reaction takes place and the hemiacetal is converted to acetal on the anomeric carbon. The carbohydrate acetals are generally called glycosides. The glycoside products in the following reaction (Fig. 9.2g) are therefore named methyl D-glucopyranoside, with α and β anomers.

The mechanism for the formation of a glycoside (Fig. 9.2h) is pretty much the same as the general mechanism from hemiacetal to acetal (section 2.5) but in a cyclic structure here.
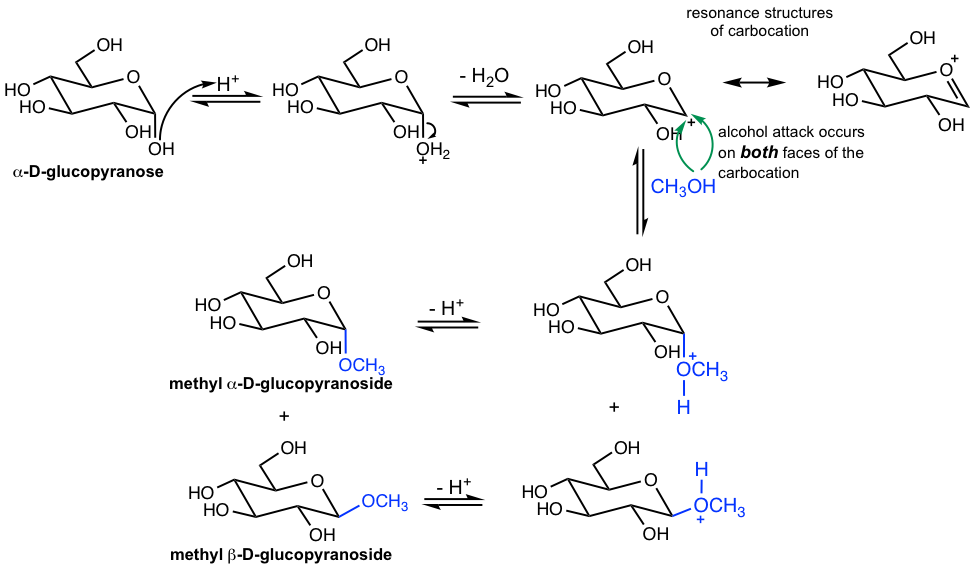
In the step of alcohol attack on the carbocation intermediate, which is stabilized by resonance effect, the attack occurs on both faces of the planar carbocation, therefore leading to the formation of both α and β anomers, even though the reactant hemiacetal is the α anomer.
Glycoside, which is an acetal, is stable in basic conditions. Glycoside therefore is used as a common protection group of the anomeric carbon in basic condition, that is to prevent undesired reactions that would involve the anomeric carbon in its hemiacetal form.
In an acidic solution, glycosides undergo hydrolysis to give back sugar and alcohol. The reaction is essentially just the hydrolysis of acetal to hemiacetal.
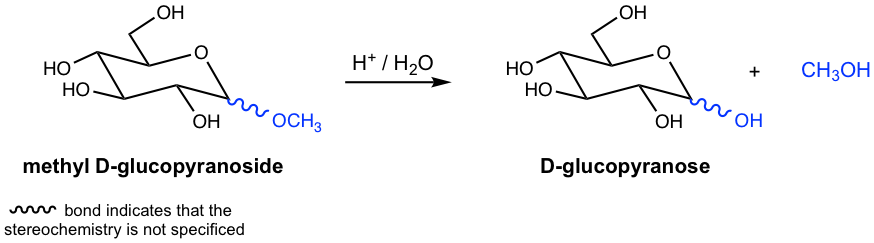